What is PGT-A (formerly called PGS)?
PGT-A, formerly called preimplantation genetic screening (PGS), is currently offered by many fertility clinics as a routine practice to screen for embryos with an incorrect number of chromosomes (aneuploidies). Screening helps better select euploid embryos (chromosomally normal embryos) for transfer and improve reproductive outcomes for IVF cycles.i Chromosome abnormalities can greatly affect embryonic development and lead to implantation failure and miscarriages. Approximately 50 percent of early pregnancy losses are due to chromosomally abnormal embryos. Often, a top-quality IVF embryo, determined by embryo grading of physical appearance, is found to be aneuploid after genetic testing.ii
PGT-A is usually considered for women with a higher risk of producing aneuploid embryos, including older women (≥35 years old) and those with a history of failed IVF cycles (recurrent implantation failure and recurrent pregnancy loss). However, several studies comparing live birth rates between women who did and did not use PGT-A have found inconsistent results, suggesting that the benefits of PGT-A are inconclusive.iii
What does PGT-A testing determine?
PGT-A is a relatively expensive technology that is also invasive, requiring the removal of embryonic cells for genetic testing.iv PGT-A cannot determine whether an embryo will implant in the uterine wall but can identify embryos with aneuploidies. Aneuploidy can lead to implantation failure, early pregnancy loss, and birth defects.v PGT-A is usually performed when embryos reach blastocyst stage, around day 5 or 6. During the procedure, about 5-10 cells are removed from the trophectoderm (TE), which are cells that will form the placenta of the blastocyst. These biopsied cells are then sent for chromosomal analysis.vi
The gold standard method for this chromosome analysis is comprehensive chromosome screening (CCS). CCS screens all 23 pairs of chromosomes, including the one pair of sex chromosomes. There are several CCS platforms available for PGT-A testing, such as next-generation sequencing (NGS), quantitative PCR (qPCR), and single-nucleotide polymorphism (SNP) array. Each method varies in terms of cost, protocol complexity, and turnaround time. PGT-A does not screen all types of genetic abnormalities and therefore does not guarantee that a live birth will not have any genetic issues. It only tests genetic abnormalities caused by issues related to chromosome numbers.
While PGT-A does not alter cumulative live birth rates, it is widely believed that PGT-A increases the live birth rate per embryo transfer for individuals undergoing IVF treatment, especially among women ≥35 years old. PGT-A will also not change the overall outcome of an IVF cycle but may quicken the time to live birth by helping avoid the transfer of aneuploid embryos. A 2020 study published in Human Reproduction analyzed 8 227 women undergoing IVF. They observed that women who used PGT-A were more likely to have a live birth after transferring good quality euploid embryos (41.7 percent) compared to women who did not use PGT-A and transferred untested good quality embryos (34.5 percent).vii
How to read PGT-A results
Table 1 shows a sample of potential chromosome analysis results from PGT-A to help understand possible outcomes and notations. Depending on the IVF laboratory in which the analysis is conducted, the format of these results will vary.
Table 1. Sample PGT-A results (see text for description)

Footnote: This is a sample of how your PGT-A results may appear, the format may vary depending on the provider. Sex may not be provided depending on location and regulations.
Euploid or “normal”
Embryo 1 in Table 1 shows how a typical euploid (“normal”) embryo biopsy result would appear in PGT-A results.
A euploid result means that there was no difference in the pattern of chromosomal material present in the sample, suggesting that all cells contain the correct number of 46 chromosomes, or 23 pairs. In most cases, each pair of chromosomes contains one chromosome from the egg and one chromosome from the sperm, though not all PGT identifies which chromosome in each pair comes from which parent.viii,ix
One of the most important factors in producing euploid embryos is the age of the maternal IVF patient or age of the egg donor. A large retrospective study published in Fertility and Sterility showed that the chance of having at least one euploid blastocyst per cycle was over 90 percent in women aged 26-37 years old.x This dropped to under 50 percent for women with advanced maternal age over 43 years old.xi These results do not account for IVF cycles where no blastocysts for testing were produced.
Based on data from three large studies, individuals under 35 years old can expect approximately 55-60 percent of their blastocyst embryos to be euploid.xii,xiii,xiv For individuals 41 years old and above, approximately 25-35 percent of their embryos are likely to euploid. It should be noted that fewer embryos make it to the testing stage in patients over 40 years old.
Aneuploid or “abnormal”
Embryo 2, 3, 4, and 5 (table 1) show how a typical aneuploid result would appear in PGT-A results. The results also specify the type of aneuploidy detected, including trisomy (Embryo 2), monosomy (Embryo 3), duplications, and deletions (embryo 5).
An aneuploid result is interpreted as an “abnormal” embryo biopsy and indicates there is a high likelihood of the sample having a missing (monosomy) or extra (trisomy) chromosome. This is most often due to an error called non-disjunction that occurs during meiosis when the egg and/or sperm are developing. Chromosomal error leading to embryo aneuploidy is much more likely to occur in the egg than in the sperm.xv,xvi
The rate of aneuploidy is approximately 40-45 percent in women under age 35 years old and approximately 65-75 percent for those 41 years and older.xvii,xviii,xix Aneuploid embryos are usually not recommended (or not permitted) for transfer, depending on the fertility clinic.xx
Trisomy
Embryo 2 in Table 1 shows an example of an embryo result of trisomy 22 (+22). The “+” indicates that there is an extra chromosome, chromosome 22, for a total of 47 chromosomes. Trisomy means there are three chromosomes in one pair, with one extra chromosome from either the sperm or the egg. Trisomy is the most common type of aneuploidy.xxi Most autosomal (non-sex chromosome) trisomies are not compatible with life, meaning that an embryo would likely not survive.
Monosomy
Embryo 3 in Table 1 shows an example of an embryo result of monosomy 7 (-7). The “-” indicates that there is a missing chromosome 7, for a total of 45 chromosomes.
Monosomy means there is a missing chromosome from either the sperm or the egg within one of the 23 chromosome pairs. Some monosomies involve the absence of a sex chromosome. For example, Turner Syndrome (45,X) is caused by the absence of an X sex chromosome. Full autosomal monosomies are not compatible with life.xxii
Complex abnormal
Embryo 4 in Table 1 shows an example of a complex abnormal result of embryo result of +3, +10, -18, -5. The “+” indicates that there is an extra chromosome 3 and an extra chromosome 10. The “-” indicates that there is a missing chromosome 5 and missing chromosome 18.
Embryo biopsy results are termed complex abnormal when there are two or more chromosome pairs affected (multiple aneuploidies). Complex abnormality is believed to result from abnormal cell division of the embryo after fertilization, as opposed to errors in the sperm or egg.xxiii Some labs also use the term chaotic abnormal to indicate five or more chromosome aneuploidies.xxiv
Duplications and deletions
Embryo 5 in Table 1 shows an example of an embryo with a duplication (dup) and a deletion (del) of a segment of a chromosome. “dup (15) (q21.2-qter)” means that a section of chromosome 5, located on the longer arm (q) of the chromosome, is duplicated or doubled. “del (6)(p24-pter)” means that a section of the short arm (p) of chromosome 6 is deleted or missing. The terms “qter” and “pter” refer to the terminus (ter) or ends of the chromosome arms.
Most PGT-A analyses can detect segmental deletions and duplications, also known as partial aneuploidies or structural abnormalities. Segmental deletions or duplications will look like a small loss or gain of genetic material in specific sections of a chromosome and can occur in any chromosome during cell division. A 2019 study published in Reproductive Biology and Endocrinology looked at PGT-A results of 3 565 blastocysts and found that segmental deletions and duplications occurred in 8.4 percent of cases.xxv
The size of the duplication or deletion that can be detected by PGT-A is usually 10-20 megabases (Mb). Sometimes smaller duplications and deletions are detectable, depending on the technique used for genetic analysis.xxvi This means that PGT-A does not detect all types of genetic duplications and deletions.
Mosaic
Embryo 6 in Table 1 shows an example of a mosaic embryo, where the result for chromosome 16 fit neither a normal nor abnormal pattern. One explanation could be the presence of an extra chromosome 16 (trisomy 16) in some cells, whereas other cells in the biopsy are euploid. In this case, the mosaicism was low-level, meaning the pattern was closer to normal than abnormal.
Chromosomal mosaicism in PGT-A could mean that the TE cells biopsied from the embryo have different genetic compositions. Usually, this means some cells were euploid and others were aneuploid.xxvii Mosaicism occurs after an embryo is fertilized due to errors as the embryo undergoes mitosis, as opposed to chromosome errors in the egg or sperm.xxviii Several recent studies have reported estimates of mosaicism ranging from 2-26 percent per blastocyst; frequency varied between clinics and techniques used.xxix
A 2020 study published in Fertility and Sterility compared IVF outcomes of PGT-A tested embryos in 1000 mosaic blastocysts and 5 561 euploid blastocysts. Researchers found that although the implantation (57.2 percent) and live birth (52.3 percent) rates were higher for euploid embryos, mosaic embryos still had an acceptable rate of implantation (46.5 percent) and live birth (37 percent).xxx Similarly, a 2019 study published in Assisted Reproduction Technologies found that mosaic embryo transfers had a live birth rate of 46.6 percent compared to 59.1 percent for euploid embryo transfers.xxxi These results suggest that patients with no available euploid embryos should receive counseling about the benefits and limitations of transferring a mosaic embryo compared to starting a new ovarian stimulation cycle.
Studies have also shown that the degree of mosaicism (i.e., low-level vs high-level mosaics) may impact potential success rates.xxxii A small study of 108 women, published in 2020 in the Journal of Clinical Medicine, showed that low-level mosaics resulted in a higher live birth rate (44.5 percent) than high-level mosaic embryos.xxxiii
The definition of low-level and high-level mosaic varies between clinics and testing labs, and different cut-off values are reported across studies. For example, low-level mosaics have been defined as anywhere from 20-50 percent of biopsied cells being aneuploid, with the rest euploid.
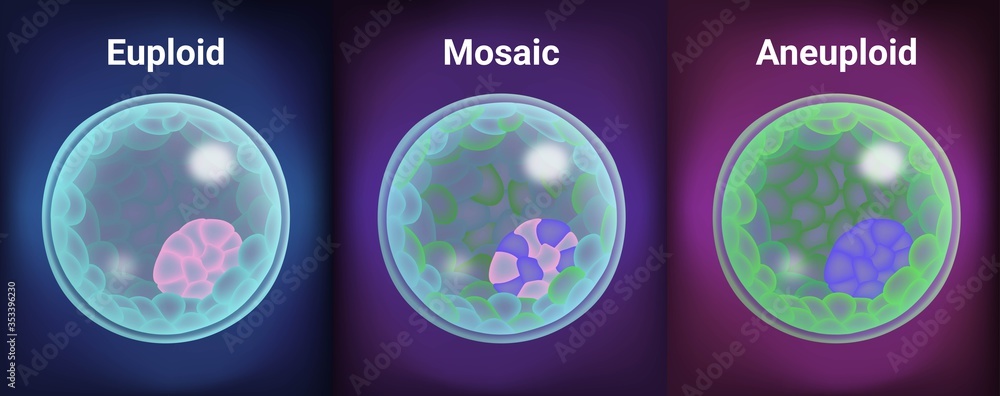
No results / inconclusive results
Embryo 7 in Table 1 shows a sample result in which the biopsy failed to yield any PGT-A results.
In approximately 2.5-2.8 percent of cases, the TE cell samples used in PGT-A yield results that are inconclusive or unavailable.xxxiv,xxxv This can occur due to errors in biopsy sampling or genetic testing. When no or inconclusive results are available, patients have the option of retesting, transferring the embryo without PGT-A results, or not using the embryo. Studies suggest that embryos with inconclusive biopsies are not more likely to be aneuploid on second biopsy so transfer without re-biopsy can be considered.xxxvi
In the case of retesting, the embryo is thawed again, another TE biopsy is taken, and the embryo is then re-frozen until the updated PGT-A results arrive. Some evidence suggests that additional biopsies and/or freeze-thaw cycles may have a negative impact on success rates.xxxvii
Triploidy
Triploidy is a chromosomal abnormality characterized by an extra set of chromosomes from either the mother or father, for a total count of 69 chromosomes. Triploidy occurs in approximately two percent of all embryos,xxxviii and a triploidy fetus can have intrauterine growth retardation (IUGR) and congenital defects. Most cases of true triploidy are not compatible with life. Not all PGT-A platforms can detect triploidy.
Sex
Embryo 1 and 5 in Table 1 show how genetically female (XX) embryos would appear in PGT-A results, while embryos 2, 3, 4, and 6 show genetically male (XY) embryos.
PGT-A not only reveals chromosome numbers but can also allow patients to know the sex of their embryos, which may affect the selection of an embryo for transfer. Sex selection of embryos using PGT-A is not permitted in many countries, including Canada and the Australian state of Victoria. In these countries, X and Y chromosome results are not revealed unless there is an abnormality. In other countries, including the United States, there are no laws against sex selection for IVF embryos following PGT-A. In these cases, “XX” or “XY” is often reported as part of the PGT results, unless requested to be masked by the patient or IVF clinic.xxxix
In addition to identifying the sex of the embryo, PGT-A can screen for aneuploidies related to an embryo's two sex chromosomes. Children born with sex chromosome aneuploidies experience a diverse range of developmental, medical, psychological, and behavioral features. Four well-known sex chromosome aneuploidies include 47,XXX females (Triple X syndrome); 47,XXY males (Klinefelter syndrome); 47,XYY males (Jacob’s syndrome); and 45,X females (Turner syndrome).xl
How accurate are PGT-A results?
In PGT-A, false positives and false negatives can occur due to biological differences in the embryo or technical or human errors during the biopsy or genetic testing process. In PGT-A, only the trophectoderm cells from blastocysts are biopsied, even though the entire blastocyst contains over 100 cells. Thus, taking a biopsy of only 5-10 TE cells may not accurately reflect the genetics of the rest of the embryo, including the embryo itself (inner cell mass).xli,xlii
Estimated PGT-A diagnostic error rates range between 2-5 percent.xliii,xliv These errors appear to be more common with mosaic embryos. A 2020 study published in Genetics compared the PGT-A results from TE and inner cell mass (ICM) biopsies of 32 blastocysts. They observed over 97 percent concordance (consistency) between TE and ICM results, but mosaic embryos only had a 35.2 percent concordance. The authors suggested that TE biopsies are less reliable for mosaic embryos.xlv
Where to get more information about PGT-A results?
Infertile couples with or without a family history of genetic diseases who are seeking IVF treatment should ask their doctor about a referral for genetic counseling. A genetic counselor can provide couples with more information about genetic testing and help them understand their PGT-A results.
In addition to helping couples make informed decisions during IVF treatment, a genetic counselor or genetic specialist may also advise couples to undergo karyotyping, which helps identify whether they are potential carriers of chromosomal abnormalities, which occur in 3-5 percent of IVF couples.xlvi
Conclusion
PGT-A testing can be a valuable screening tool for women undergoing IVF, especially if individuals have risk factors that increase their likelihood of producing embryos with an incorrect number of chromosomes. These risk factors, including a history of failed IVF cycles, recurrent pregnancy loss, and advanced maternal age, should be discussed with the individual’s or couple’s medical team as they make informed decisions about their assisted reproduction options.
i Chen, H., et al. (2020). An overview of the current and emerging platforms for preimplantation genetic testing for aneuploidies (PGT-A) in in vitro fertilization programs. Taiwanese Journal of Obstetrics and Gynecology, 59(4), 489-495. https://doi.org/10.1016/j.tjog.2020.05.004
ii Fesahat, F., et al. (2020). Preimplantation genetic testing in assisted reproduction technology. Journal of Gynecology Obstetrics and Human Reproduction, 49(5), 101723. https://doi.org/10.1016/j.jogoh.2020.101723
iii Haviland, M. J., et al. (2020). Comparison of pregnancy outcomes following preimplantation genetic testing for aneuploidy using a matched propensity score design. Human Reproduction, 35(10), 2356-2364. https://doi.org/10.1093/humrep/deaa161
iv Haviland, M. J., et al. (2020). Comparison of pregnancy outcomes following preimplantation genetic testing for aneuploidy using a matched propensity score design. Human Reproduction, 35(10), 2356-2364. https://doi.org/10.1093/humrep/deaa161
v Fesahat, F., et al. (2020). Preimplantation genetic testing in assisted reproduction technology. Journal of Gynecology Obstetrics and Human Reproduction, 49(5), 101723. https://doi.org/10.1016/j.jogoh.2020.101723
vi Popovic, M., et al. (2020). Erratum. Chromosomal mosaicism in human blastocysts: The ultimate diagnostic dilemma. Human Reproduction Update, 26(3), 450-451. https://doi.org/10.1093/humupd/dmaa015
vii Haviland, M. J., et al. (2020). Comparison of pregnancy outcomes following preimplantation genetic testing for aneuploidy using a matched propensity score design. Human Reproduction, 35(10), 2356-2364. https://doi.org/10.1093/humrep/deaa161
viii Popovic, M., et al. (2020). Erratum. Chromosomal mosaicism in human blastocysts: The ultimate diagnostic dilemma. Human Reproduction Update, 26(3), 450-451. https://doi.org/10.1093/humupd/dmaa015
ix Shahbazi, M. N., et al. (2020). Developmental potential of aneuploid human embryos cultured beyond implantation. Nature Communications, 11(1). https://doi.org/10.1038/s41467-020-17764-7
x Franasiak, J. M., et al. (2014). The nature of aneuploidy with increasing age of the female partner: A review of 15,169 consecutive trophectoderm biopsies evaluated with comprehensive chromosomal screening. Fertility and Sterility, 101(3), 656-663.e1. https://doi.org/10.1016/j.fertnstert.2013.11.004
xi Franasiak, J. M., et al. (2014). The nature of aneuploidy with increasing age of the female partner: A review of 15,169 consecutive trophectoderm biopsies evaluated with comprehensive chromosomal screening. Fertility and Sterility, 101(3), 656-663.e1. https://doi.org/10.1016/j.fertnstert.2013.11.004
xii Franasiak, J. M., et al. (2014). The nature of aneuploidy with increasing age of the female partner: A review of 15,169 consecutive trophectoderm biopsies evaluated with comprehensive chromosomal screening. Fertility and Sterility, 101(3), 656-663.e1. https://doi.org/10.1016/j.fertnstert.2013.11.004
xiii Demko, Z. P., et al. (2016). Effects of maternal age on euploidy rates in a large cohort of embryos analyzed with 24-chromosome single-nucleotide polymorphism–based preimplantation genetic screening. Fertility and Sterility, 105(5), 1307-1313. https://doi.org/10.1016/j.fertnstert.2016.01.025
xiv Rubio, C., et al. (2019). Clinical application of embryo aneuploidy testing by next-generation sequencing. Biology of Reproduction, 101(6), 1083-1090. https://doi.org/10.1093/biolre/ioz019
xv Kubicek, D., et al. (2019). Incidence and origin of meiotic whole and segmental chromosomal aneuploidies detected by karyomapping. Reproductive BioMedicine Online, 38(3), 330-339. https://doi.org/10.1016/j.rbmo.2018.11.023
xvi Templado, C., et al. (2011). Aneuploidy in human spermatozoa. Cytogenetic and Genome Research, 133(2-4), 91-99. https://doi.org/10.1159/000323795
xvii Demko, Z. P., et al. (2016). Effects of maternal age on euploidy rates in a large cohort of embryos analyzed with 24-chromosome single-nucleotide polymorphism–based preimplantation genetic screening. Fertility and Sterility, 105(5), 1307-1313. https://doi.org/10.1016/j.fertnstert.2016.01.025
xviii Franasiak, J. M., et al. (2014). The nature of aneuploidy with increasing age of the female partner: A review of 15,169 consecutive trophectoderm biopsies evaluated with comprehensive chromosomal screening. Fertility and Sterility, 101(3), 656-663.e1. https://doi.org/10.1016/j.fertnstert.2013.11.004
xix Rubio, C., et al. (2019). Clinical application of embryo aneuploidy testing by next-generation sequencing. Biology of Reproduction, 101(6), 1083-1090. https://doi.org/10.1093/biolre/ioz019
xx Shahbazi, M. N., et al. (2020). Developmental potential of aneuploid human embryos cultured beyond implantation. Nature Communications, 11(1). https://doi.org/10.1038/s41467-020-17764-7
xxi Soler, A., et al. (2017). Overview of chromosome abnormalities in first trimester miscarriages: A series of 1,011 consecutive chorionic villi sample karyotypes. Cytogenetic and Genome Research, 152(2), 81-89. https://doi.org/10.1159/000477707
xxii Viotti, M. (2020). Preimplantation genetic testing for chromosomal abnormalities: Aneuploidy, mosaicism, and structural rearrangements. Genes, 11(6), 602. https://doi.org/10.3390/genes11060602
xxiii Voullaire, L., et al. (2007). High incidence of complex chromosome abnormality in cleavage embryos from patients with repeated implantation failure. Fertility and Sterility, 87(5), 1053-1058. https://doi.org/10.1016/j.fertnstert.2006.11.043
xxiv Rubio, C., et al. (2019). Clinical application of embryo aneuploidy testing by next-generation sequencing. Biology of Reproduction, 101(6), 1083-1090. https://doi.org/10.1093/biolre/ioz019
xxv Escribà, M., et al. (2019). Segmental aneuploidy in human blastocysts: A qualitative and quantitative overview. Reproductive Biology and Endocrinology, 17(1). https://doi.org/10.1186/s12958-019-0515-6
xxvi Escribà, M., et al. (2019). Segmental aneuploidy in human blastocysts: A qualitative and quantitative overview. Reproductive Biology and Endocrinology, 17(1). https://doi.org/10.1186/s12958-019-0515-6
xxvii Popovic, M., et al. (2020). Erratum. Chromosomal mosaicism in human blastocysts: The ultimate diagnostic dilemma. Human Reproduction Update, 26(3), 450-451. https://doi.org/10.1093/humupd/dmaa015
xxviii Spinella, F., et al. (2017). The extent of chromosomal mosaicism influences the clinical outcome of in vitro fertilization treatments. Fertility and Sterility, 108(3), e272. https://doi.org/10.1016/j.fertnstert.2017.07.809
xxix Popovic, M., et al. (2020). Erratum. Chromosomal mosaicism in human blastocysts: The ultimate diagnostic dilemma. Human Reproduction Update, 26(3), 450-451. https://doi.org/10.1093/humupd/dmaa015
xxx Viotti, M., et al. (2021). Using outcome data from one thousand mosaic embryo transfers to formulate an embryo ranking system for clinical use. Fertility and Sterility, 115(5), 1212-1224. https://doi.org/10.1016/j.fertnstert.2020.11.041
xxxi Zhang, L., et al. (2018). Rates of live birth after mosaic embryo transfer compared with euploid embryo transfer. Journal of Assisted Reproduction and Genetics, 36(1), 165-172. https://doi.org/10.1007/s10815-018-1322-2
xxxii Spinella, F., et al. (2017). The extent of chromosomal mosaicism influences the clinical outcome of in vitro fertilization treatments. Fertility and Sterility, 108(3), e272. https://doi.org/10.1016/j.fertnstert.2017.07.809
xxxiii Lin, P., et al. (2020). Clinical outcomes of single mosaic embryo transfer: High-level or low-level mosaic embryo, does it matter? Journal of Clinical Medicine, 9(6), 1695. https://doi.org/10.3390/jcm9061695
xxxiv Franasiak, J. M., et al. (2014). The nature of aneuploidy with increasing age of the female partner: A review of 15,169 consecutive trophectoderm biopsies evaluated with comprehensive chromosomal screening. Fertility and Sterility, 101(3), 656-663.e1. https://doi.org/10.1016/j.fertnstert.2013.11.004
xxxv Cimadomo, D., et al. (2018). Inconclusive chromosomal assessment after blastocyst biopsy: Prevalence, causative factors and outcomes after re-biopsy and re-vitrification. A multicenter experience. Human Reproduction, 33(10), 1839-1846. https://doi.org/10.1093/humrep/dey282
xxxvi Cimadomo, D., et al. (2018). Inconclusive chromosomal assessment after blastocyst biopsy: Prevalence, causative factors and outcomes after re-biopsy and re-vitrification. A multicenter experience. Human Reproduction, 33(10), 1839-1846. https://doi.org/10.1093/humrep/dey282
xxxvii Bradley, C. K., et al. (2017). Impact of multiple blastocyst biopsy and vitrification-warming procedures on pregnancy outcomes. Fertility and Sterility, 108(6), 999-1006. https://doi.org/10.1016/j.fertnstert.2017.09.013
xxxviii Witters, G., et al. (2011). Trisomy 13, 18, 21, Triploidy and Turner syndrome: the 5T's. Look at the hands. Facts, views & vision in ObGyn, 3(1), 15–21.
xxxix Kovacs, G. (2013). Should couples be allowed to select the sex of their offspring? Journal of Obstetrics and Gynaecology Canada, 35(12), 1105-1107. https://doi.org/10.1016/s1701-2163(15)30761-1
xl Hall, H., et al. (2006). Meiosis and sex chromosome aneuploidy: How meiotic errors cause aneuploidy; how aneuploidy causes meiotic errors. Current Opinion in Genetics & Development, 16(3), 323-329. https://doi.org/10.1016/j.gde.2006.04.011
xli Chan, C., et al. (2021). Preimplantation genetic testing for aneuploidy: A Canadian fertility and Andrology society guideline. Reproductive BioMedicine Online, 42(1), 105-116. https://doi.org/10.1016/j.rbmo.2020.10.020
xlii Viotti, M. (2020). Preimplantation genetic testing for chromosomal abnormalities: Aneuploidy, mosaicism, and structural rearrangements. Genes, 11(6), 602. https://doi.org/10.3390/genes11060602
xliii Bellver, J., et al. (2019). undefined. Reproductive BioMedicine Online, 39(6), 905-915. https://doi.org/10.1016/j.rbmo.2019.07.037
xliv Neal, S. A., et al. (2018). Preimplantation genetic testing for aneuploidy is cost-effective, shortens treatment time, and reduces the risk of failed embryo transfer and clinical miscarriage. Fertility and Sterility, 110(5), 896-904. https://doi.org/10.1016/j.fertnstert.2018.06.021
xlv Sachdev, N. M., et al. (2021). The reproducibility of Trophectoderm biopsies in Euploid, aneuploid, and mosaic embryos using independently verified next-generation sequencing (NGS): A pilot study. Obstetrical & Gynecological Survey, 76(8), 482-483. https://doi.org/10.1097/01.ogx.0000791692.55762.1b
xlvi Coco, R. (2018). Genetic counseling prior to assisted reproductive technology procedures in the era of cytogenomics. JBRA Assisted Reproduction, 22(4), 375-380. https://doi.org/10.5935/1518-0557.20180050