What is DNA?
Deoxyribonucleic acid (DNA) is a molecule that contains the blueprint for making the proteins in an individual human body. One way to think of DNA is that it is essentially an instruction manual that, when read, lays out how an individual human being is formed. Each individual has DNA that is unique to them and that is inherited equally from the sperm and egg that made them. One exception is in the case of identical twins, who share the same DNA/genetic make-up.
DNA consists of two long strands that weave together in the form of a double helix which looks like a spiral staircase. Each of the two strands is formed by a sequence of four basic building blocks called nucleotides, and each nucleotide contains one of four bases - adenine (A), cytosine (C), guanine (G) and thymine (T). Every base is paired with a matching base on the opposite strand.
The four bases are ordered in different 3-base sequences along the DNA strands, with each 3-base sequence coding for a different amino acid. Amino acids are the fundamental building blocks of proteins. The order of the base sequences determines the protein makeup of an individual; the variability of these base sequences and the order in which they appear are what make each individual unique.
A gene is a specific section of DNA that codes for a particular molecule, usually a protein. Genes contribute to all the individual traits that people possess, from eye color to the propensity to acquire specific genetic illnesses or chronic health conditions. The genome is the complete selection of genes that an organism possesses. It is still unknown exactly how many genes are in the human genome; however, the most recent estimates suggest there are over 21 000 genes.i Each gene is in a specific site on a specific chromosome and each chromosome holds the code for many genes.
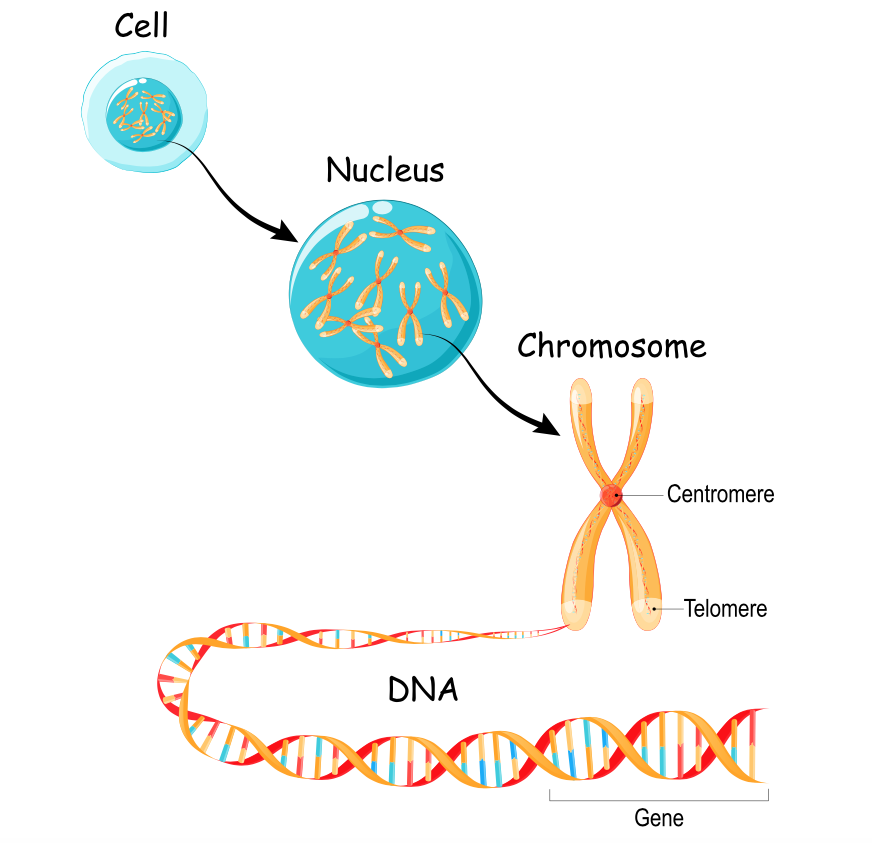
If a single strand of DNA was uncoiled in a straight line, each strand would measure around 6.5 feet and the entirety of the DNA in a single human body would measure twice the diameter of the solar system - 67 billion miles long. Thus, in order to contain all the information required to form a full human being, DNA is exceptionally tightly coiled.ii
Not only is DNA structured as two strands wrapping around each other, but each of these double helices is also further coiled many times over to form a structure called a chromosome. This is the only way the vast amount of genetic material required to build a human could fit into each individual cell in the body.
How many chromosomes are there in human cells?
Most people have their DNA stored as 23 pairs of chromosomes for a total of 46 chromosomes. It is worth noting that some people have genetic conditions where they have a different number of total chromosomes than the expected number of 46.
Of the 46 chromosomes, 44 are called autosomes (simply meaning they are not sex chromosomes). These 22 pairs of autosomes are numbered from 1 to 22, roughly based on their size from largest to smallest. The remaining two chromosomes are sex chromosomes, which can be either X or Y. These chromosomes determine the biological sex of a human being. Biological sex is also known as natal sex or birth sex. Most natal females have two X sex chromosomes (XX), while most natal males have one X and one Y sex chromosome (XY). Putting this together, most natal female human beings are 46XX and natal male human beings are 46XY.
A karyotype is the total array of chromosomes an individual possesses, and karyotyping is the laboratory technique used to take a picture of these chromosomes for chromosome analysis or to look for missing or extra chromosomes or differences in the structure of the chromosomes.
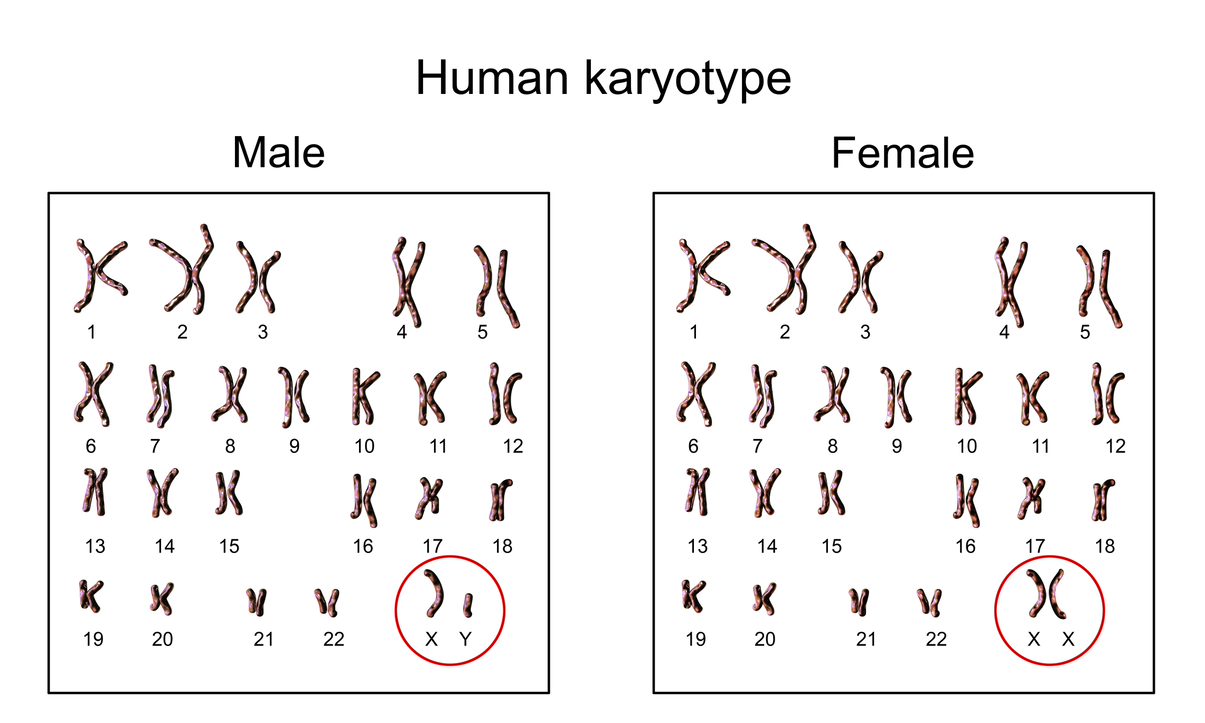
Scientists who study genetics (known as geneticists) have been able to determine the exact chromosomal location for most genes, which can be documented almost like an address. For example, a gene that increases risk for kidney cancer has been localized to 3p25.3, meaning on the 3rd chromosome, on the p-arm (short arm), band 2, sub-band 5, and sub-sub-band 3. This gives the exact location on the chromosome for this specific gene.iii
At which developmental stages can chromosome testing be done?
Genetic testing can be performed at many different stages of pregnancy, as well as before a couple is even trying to conceive.
Helpful terminology to know regarding chromosome abnormalities:
- Aneuploidy (adjective: aneuploid): a condition where there are either extra chromosomes or missing chromosomes; in this case, the total chromosome number is not 46
- Euploidy (adjective: euploid): having the full set of 46 chromosomes seen in most human beings
- Chromosomal Mosaicism (adjective: mosaic): a condition where one person’s cells do not all contain the same DNA; different cells in the person’s body contain different genetic codes; the level of mosaicism (high vs low or a percentage) indicates the number of cells with abnormal genetics
Below is a look at the different types of chromosome analyses that may be done and at what stages the chromosomal material can be examined.
Preimplantation genetic testing (PGT)
Preimplantation Genetic Testing (PGT) is a collection of genetic tests that can be performed on an embryo during IVF prior to the transfer of an embryo into the uterus.iv PGT can both be used to screen embryos for known genetic illnesses in the families of one or both parents, and for random chromosomal issues that may have begun when the embryo started to develop in the lab.
There are three broad categories of PGT: PGT for aneuploidy (PGT-A), PGT for monogenic diseases (PGT-M), and PGT for structural chromosome rearrangements (PGT-SR). PGT-A is the type of genetic testing that involves chromosomes with the incorrect number of chromosomes, called aneuploidy.
A full description of the nature of PGT is outside the scope of this article. For additional information, please see this related article.
Prenatal Screening
Prenatal screening is often performed starting in the first trimester of pregnancy to determine the risk of a fetus having certain genetic or congenital disorders. This type of screening uses a parental blood sample and does not carry any risk to the pregnancy/fetus.
What is the difference between prenatal screening and prenatal diagnostic testing (PDT)?
It is important to distinguish prenatal screening from prenatal diagnostic testing (PDT). PDT offers ways of definitively diagnosing a fetus with a specific genetic condition through invasive methods which carry a small risk of fetal loss. PDT includes chorionic villus sample (CVS) and amniocentesis (“amnio”). This is in comparison to prenatal screening, which does not definitively diagnose a fetus with a specific genetic condition but gives information about the risk for such conditions. In most cases, if a genetic condition is suspected based on prenatal screening, CVS or amnio is recommended to get a definitive diagnosis.
Prenatal screening typically consists of a maternal blood test with or without specific fetal ultrasound findings. ACOG recommends that all pregnant women, regardless of maternal age or risk for miscarriage or for carrying a genetic condition, be offered prenatal screening. They also state that after considering the risks and benefits of such testing, pregnant individuals should decide themselves whether to pursue this testing or not.v
Prenatal cell-free DNA (cfDNA) screening
The current gold standard for prenatal screening is prenatal cell-free DNA (cfDNA, also referred to as non-invasive prenatal screening or testing, NIPS or NIPT) testing (e.g., Harmony, Panorama, Verifi, Invitae NIPS). A small amount of DNA (from the placenta) is found in the blood stream of a pregnant woman. During pregnancy, DNA from the placenta is released into the maternal bloodstream as cells die off. The DNA of the placenta is typically identical to the DNA of the fetus. Although the exact method changes from lab to lab, cfDNA screening analyzes the cell free DNA in the blood (both maternal and placental) for an indication of missing or extra material from certain chromosomes. If an abnormality is detected, this may increase the risk of the pregnancy having a certain chromosomal condition. The cfDNA screening can be performed after 9 to 10 weeks' gestation.
The cfDNA extracted from the maternal blood is tested for specific aneuploidies, including trisomy 21 (Down syndrome), trisomy 13, and trisomy 18, and sex chromosome aneuploidy (including Turner and Klinefelter syndrome). In one recent study of 15 841 women who underwent cfDNA testing between 10 to 14 weeks of gestation, fetal trisomy 21 was correctly identified in all 38 women whose offspring were born with trisomy 21. The cfDNA test also correctly identified 9 of 10 cases of trisomy 18 and 2 of 2 cases of trisomy 13. The false positive rate was only 0.06 percent, meaning that in 0.06 percent of cases, cfDNA identified an aneuploidy when there was not one.vi In the case of twins, cfDNA can still be used. However, because of the rarity of both aneuploidy and twin pregnancy, NIPT accuracy in twin pregnancy is less clear.vii,viii Some NIPTs also screen for structural changes in chromosomes, such as microdeletions (e.g., Prader-Willi, DiGeorge, and Cri-Du-Chat syndromes), where a small portion of a certain chromosome is missing.ix However, the screening for microdeletions is newer, more challenging, and considered less reliable than for whole chromosome aneuploidies (where an entire chromosome is missing or gained).x,xi,xii This is in part due to the low frequency of specific microdeletions, and thus the lack of clinical validation datasets to ensure test reliability. As such, some companies do not offer microdeletions or other structural chromosome abnormalities in their screening panels.
For NIPTs, patients should remember that these are screening tests and not diagnostic. As described above, positive screening results should be followed up with genetic counseling, ultrasound assessment, and offering of CVS or amniocentesis for a reliable diagnosis.xiii,xiv It is usually not recommended to make decisions about a pregnancy based solely on screening results, due to potential inaccuracies such as false positive results.
Nuchal translucency ultrasound testing
Nuchal translucency may be used alone or in combination with maternal serum screening for prenatal screening in the first trimester. This test involves an ultrasound at 11w-13w6d gestation, which, in combination with bloodwork, assesses the risk of fetal aneuploidy.
Nuchal translucency is the appearance on ultrasound of fluid under the skin of the fetal neck; fetuses with more fluid are more likely to have a case of aneuploidy, such as trisomy 21 or Turner syndrome. Some studies have shown that if both a nuchal translucency scan and cfDNA are used, accuracy is improved.xv,xvi NACOG recommends that if enlarged nuchal translucency (excess clear fluid) is seen at 11-14 weeks, patients should be given genetic counseling and offered 18–22 week anatomic ultrasound and amniocentesis or CVS.
Other prenatal screening methods
There are several older methods to screen for risk of fetal aneuploidy, all of which are usually performed using the nuchal translucency measurement and/or a blood sample drawn from the pregnant woman:
- First trimester screen: consists of an ultrasound to measure nuchal translucency as well as maternal blood testing for levels of Papp-A and hCG.xvii
- Quad screen: consists of a maternal blood test performed between 15 and 22 weeks of pregnancy which measures levels of four different substances: alpha-fetoprotein (AFP), hCG (human chorionic gonadotropin), estriol, and inhibin-A.xviii
- Triple screen: a test similar to the quad screen, which measures all the markers in quad screen except inhibin-A.xix
- Integrated screen: the results of the first trimester nuchal translucency measurement and PAPP-A level are considered in combination with second trimester quad screen to create a risk profile for risk of aneuploidy.xx
Table 1. Prenatal Screening Methods
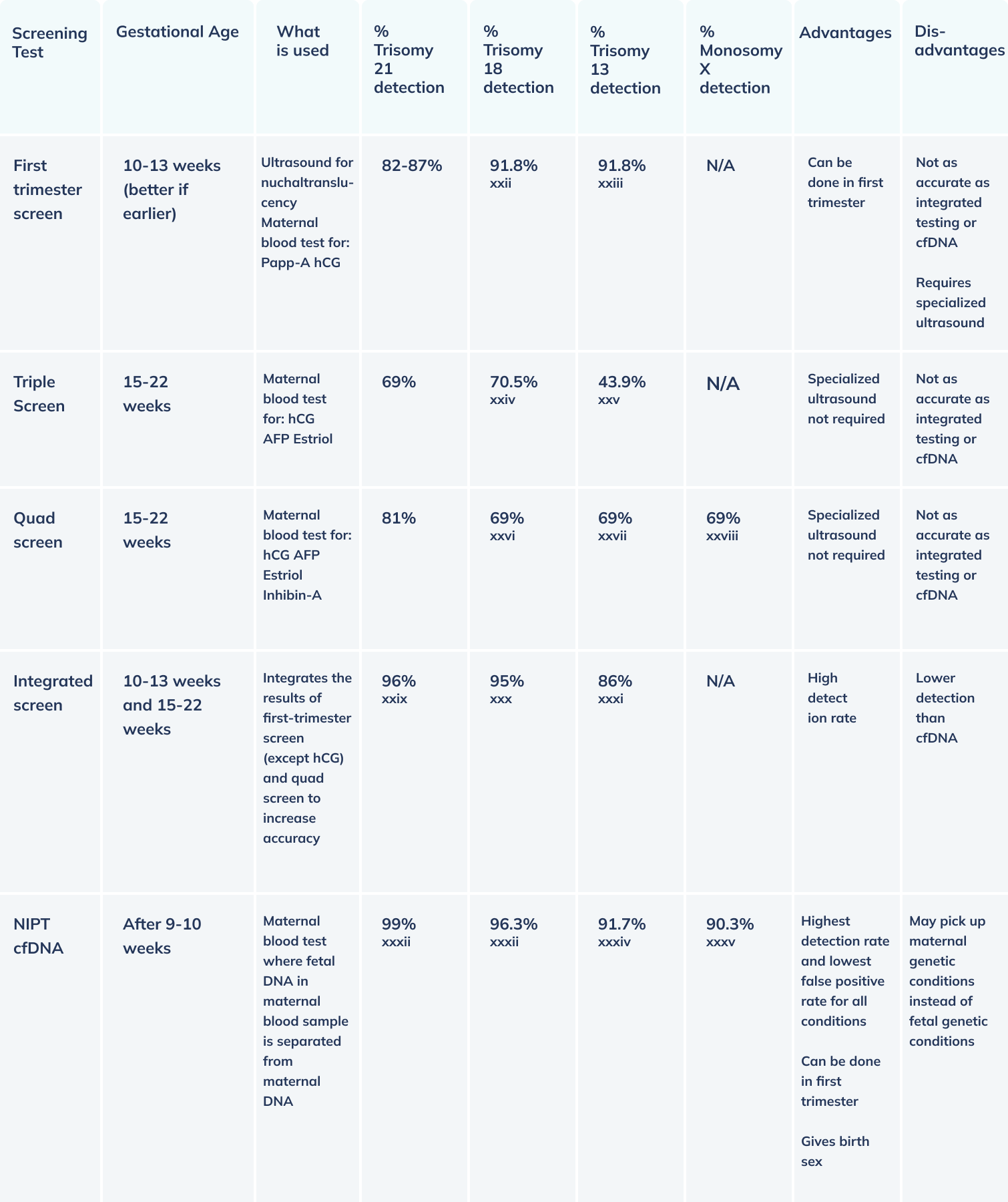
Prenatal Diagnostic Testing (PDT) for Genetic Disorders
If there is a concern for a genetic condition based on either PGT or prenatal screening, prenatal diagnostic testing (PDT) may be recommended.
ACOG recommends prenatal diagnostic testing be offered to all women regardless of age or risk factors, but the risks and benefits should be discussed based on an individual patient’s risk factors for fetal genetic issues. In some of the following situations, individuals may be encouraged to pursue PDT: older maternal or paternal age, parents who are carriers of/ have chromosomal rearrangements or aneuploidy, those with a prior child with structural birth defects or who are known carriers of genetic disorders, or if there are possible anomalies seen on ultrasound.xxxviii
In order to perform prenatal diagnostic testing, two different procedures are available during pregnancy. These are referred to as CVS and amniocentesis. In both cases, medical providers obtain tissue samples to send to a lab for definitive genetic testing. Depending on the indication, multiple tests might be offered using the sample obtained from CVS or amniocentesis. Karyotyping is a type of chromosome analysis that provides a picture of all the chromosomes in the fetus to assess the number and structure of chromosomes. Fluorescence in situ hybridization analysis (FISH), chromosomal microarrays, and whole genome (or exome) sequencing are other genetic techniques that also test for the presence of chromosomal abnormalities.xxxix,xl
Karyotypes, FISH, microarray, and sequencing techniques can accurately identify aneuploidies, e.g., monosomies and trisomies. However, none of these tests will detect all genetic conditions,xli including conditions caused by single-gene abnormalities. Karyotyping has a detection rate of >99 percent for aneuploidies and large chromosomal problems.xlii
Chorionic villous sampling (CVS)
CVS can be performed between 10 and 13 weeks of gestation.xliii CVS is a procedure during which a sample of placental villi (fingerlike projections on the placenta that allow transport of nutrients from the placenta to the fetus) is obtained with a needle or catheter passed under ultrasound guidance through the abdomen or cervix.xliv
CVS is considered to be safe. A study by Akolekar et al (2015) of 8 899 women undergoing CVS found that the chance of pregnancy loss was only 0.22 percent.xlv There is a theoretical risk of fetal limb loss with CVS, but this appears to be extremely rare and mostly associated with CVS before 10 weeks.xlvi
Side effects experienced after CVS include mild vaginal bleeding (in 32 percent of patients if the procedure is performed through the cervix). Other risks such as failure to get a sample, leakage of amniotic fluid, or intrauterine infection are very low – less than 0.5 percent.xlvii
Amniocentesis
Amniocentesis is typically performed between 15 and 20 weeks of gestation but can be done at any later gestational age as well.xlviii A physician performing an amniocentesis places a small, long, sterile needle through the abdomen and into the amniotic sac under ultrasound guidance.xlix Approximately 20-30mL of amniotic fluid is aspirated through the needle.l
The risk of pregnancy loss with amniocentesis is approximately 0.11 percent, and other possible adverse effects that include vaginal spotting or leakage of amniotic fluid occur in 1-2 percent of cases.li,lii Risks of needle injury to the fetus or diagnostic failure are exceedingly low.liii
Table 2. Prenatal Diagnostic Tests
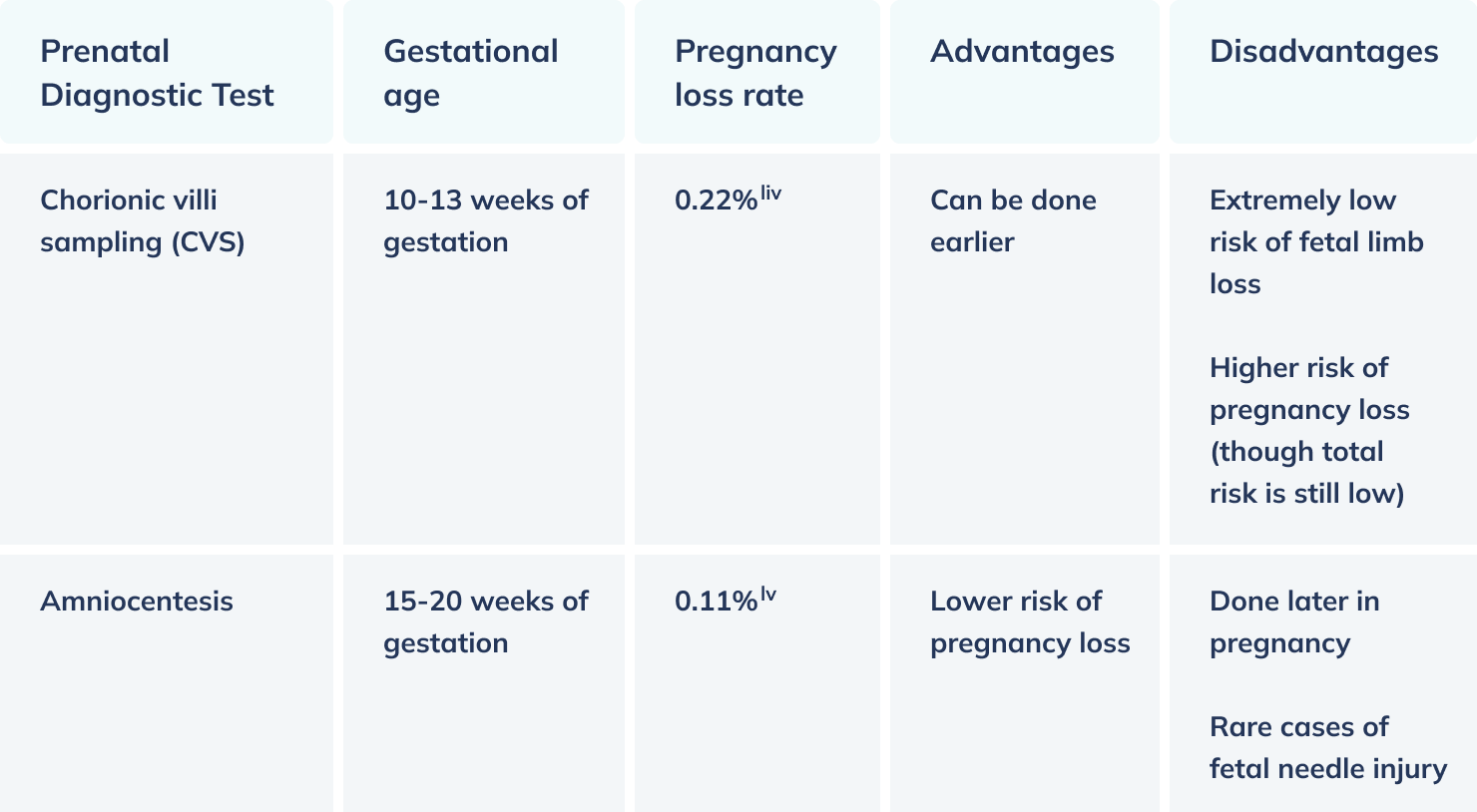
What outcomes are associated with chromosome abnormalities?
The table below shows what outcomes may be associated with various chromosomal abnormalities, delineated by chromosomal syndrome name, features, life expectancy, and frequency.
Table 3. Outcomes associated with various chromosomal abnormalities, delineated bychromosomal syndrome name, features, life expectancy, and frequency
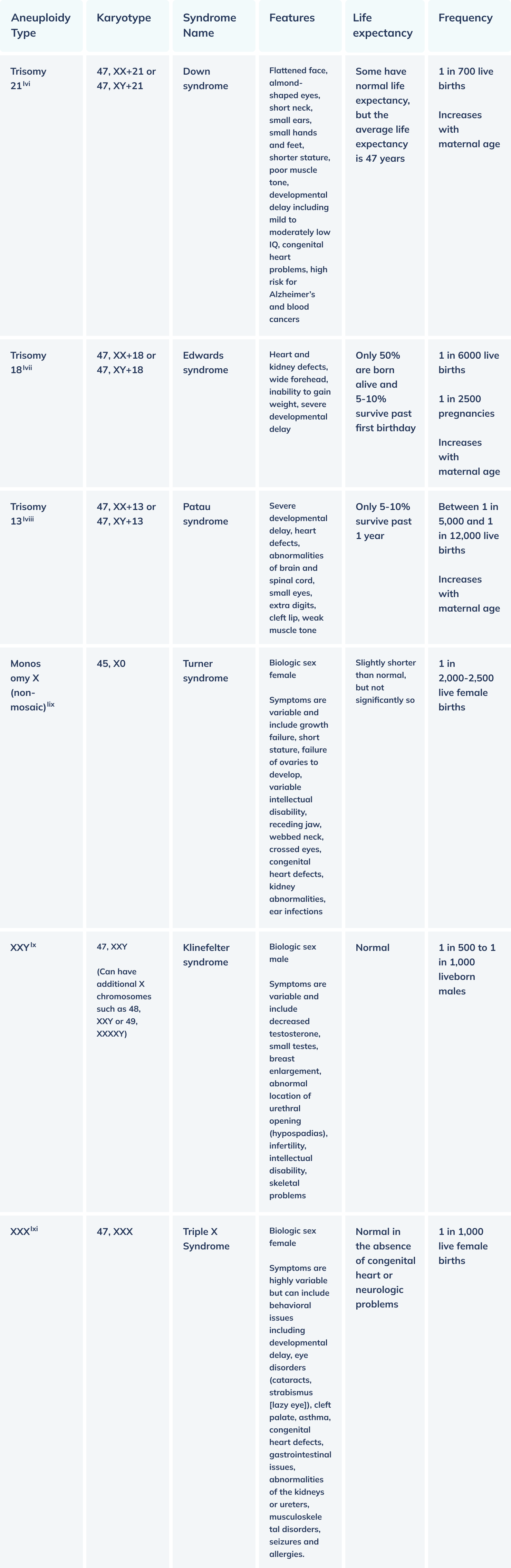
Conclusion
In sum, chromosome analysis offers a closer look at what could be leading to recurrent miscarriage and what else could potentially affect the fetus. Medical genetics is a growing body of research, and the clinical significance of chromosome analysis has grown in recent years. Anyone interested in genetic testing or chromosome analysis should speak to their healthcare provider for more information.
i Willyard, C. (2018). New human gene tally reignites debate. Nature, 558(7710), 354-355. https://doi.org/10.1038/d41586-018-05462-w
ii Toledo, C., & Saltsman, K. (2012). Genetics by the numbers. National Institute of General Medical Sciences. https://www.nigms.nih.gov/education/Inside-Life-Science/Pages/genetics-by-the-numbers.aspx
iii Büscheck, F., et al. (2020). Prevalence and clinical significance of VHL mutations and 3p25 deletions in renal tumor subtypes. Oncotarget, 11(3), 237-249. https://doi.org/10.18632/oncotarget.27428
iv Preimplantation genetic testing. (2020). Obstetrics & Gynecology, 135(3), e133-e137. https://doi.org/10.1097/aog.0000000000003714
v The American College of Obstetricians and Gynecologists. (n.d.). Current ACOG guidance. https://www.acog.org/advocacy/policy-priorities/non-invasive-prenatal-testing/current-acog-guidance
vi Norton, M. E., et al. (2015). Cell-free DNA analysis for Noninvasive examination of trisomy. Obstetrical & Gynecological Survey, 70(8), 483-484. https://doi.org/10.1097/01.ogx.0000470657.58577.f2
vii The American College of Obstetricians and Gynecologists. (n.d.). Current ACOG guidance. https://www.acog.org/advocacy/policy-priorities/non-invasive-prenatal-testing/current-acog-guidance
viii Gil, M. M., et al. (2019). Screening for Trisomies by cfDNA testing of maternal blood in twin pregnancy: Update of the fetal medicine Foundation results and meta-analysis. Obstetrical & Gynecological Survey, 74(11), 627-629. https://doi.org/10.1097/ogx.0000000000000740
ix Kucharik, M., et al. (2020). Non-invasive prenatal testing (NIPT) by low coverage genomic sequencing: Detection limits of screened chromosomal microdeletions. PLOS ONE, 15(8), e0238245. https://doi.org/10.1371/journal.pone.0238245
x Hu, Y., et al. (2022). Clinical utility of expanded NIPT for chromosomal abnormalities and etiology analysis of cytogenetic discrepancies cases. Journal of Assisted Reproduction and Genetics. https://doi.org/10.1007/s10815-021-02351-6
xi Zhao, C., et al. (2015). Detection of fetal Subchromosomal abnormalities by sequencing circulating cell-free DNA from maternal plasma. Clinical Chemistry, 61(4), 608-616. https://doi.org/10.1373/clinchem.2014.233312
xii Harraway J. (2017). Non-invasive prenatal testing. Australian family physician, 46(10), 735–739.
xiii The American College of Obstetricians and Gynecologists. (n.d.). Current ACOG guidance. https://www.acog.org/advocacy/policy-priorities/non-invasive-prenatal-testing/current-acog-guidance
xiv Harraway J. (2017). Non-invasive prenatal testing. Australian family physician, 46(10), 735–739.
xv The Fetal Medicine Foundation. (n.d.). Nuchal translucency scan. https://fetalmedicine.org/fmf-certification-2/nuchal-translucency-scan
xvi Kagan, K. O., et al. (2018). First-trimester risk assessment based on ultrasound and cell-free DNAvscombined screening: A randomized controlled trial. Ultrasound in Obstetrics & Gynecology, 51(4), 437-444. https://doi.org/10.1002/uog.18905
xvii Wright, D., et al. (2014). First-trimester screening for Trisomies 21, 18 and 13 by ultrasound and biochemical testing. Fetal Diagnosis and Therapy, 35(2), 118-126. https://doi.org/10.1159/000357430
xviii Carlson, L. M., & Vore, N. L. (2017). Prenatal diagnosis: Screening and diagnostic tools. Obstetrics and Gynecology Clinics of North America, 44(2), 245-256. https://doi.org/10.1016/j.ogc.2017.02.004
xix Metcalfe, A., et al. (2014). Beyond trisomy 21: Additional chromosomal anomalies detected through routine Aneuploidy screening. Journal of Clinical Medicine, 3(2), 388-415. https://doi.org/10.3390/jcm3020388
xx Bestwick, J. P., & Wald, N. J. (2015). Antenatal screening for down’s syndrome, trisomy 18, and trisomy 13: Reporting a single screening result for all three. Journal of Medical Screening, 22(2), 100-105. https://doi.org/10.1177/0969141315575545
xxi Malone, F. D., et al. (2005). First-trimester or second-trimester screening, or both, for down's syndrome. The New England Journal of Medicine. https://doi.org/10.1056/NEJMoa043693
xxii Wright, D., et al. (2014). First-trimester screening for Trisomies 21, 18 and 13 by ultrasound and biochemical testing. Fetal Diagnosis and Therapy, 35(2), 118-126. https://doi.org/10.1159/000357430
xxiii Wright, D., et al. (2014). First-trimester screening for Trisomies 21, 18 and 13 by ultrasound and biochemical testing. Fetal Diagnosis and Therapy, 35(2), 118-126. https://doi.org/10.1159/000357430
xxiv Metcalfe, A., et al. (2014). Beyond trisomy 21: Additional chromosomal anomalies detected through routine Aneuploidy screening. Journal of Clinical Medicine, 3(2), 388-415. https://doi.org/10.3390/jcm3020388
xxv Metcalfe, A., et al. (2014). Beyond trisomy 21: Additional chromosomal anomalies detected through routine Aneuploidy screening. Journal of Clinical Medicine, 3(2), 388-415. https://doi.org/10.3390/jcm3020388
xxvi Breathnach, F. M., et al. (2007). First- and second-trimester screening. Obstetrics & Gynecology, 110(3), 651-657. https://doi.org/10.1097/01.aog.0000278570.76392.a6
xxvii Breathnach, F. M., et al. (2007). First- and second-trimester screening. Obstetrics & Gynecology, 110(3), 651-657. https://doi.org/10.1097/01.aog.0000278570.76392.a6
xxviii Breathnach, F. M., et al. (2007). First- and second-trimester screening. Obstetrics & Gynecology, 110(3), 651-657. https://doi.org/10.1097/01.aog.0000278570.76392.a6
xxix Bestwick, J. P., & Wald, N. J. (2015). Antenatal screening for down’s syndrome, trisomy 18, and trisomy 13: Reporting a single screening result for all three. Journal of Medical Screening, 22(2), 100-105. https://doi.org/10.1177/0969141315575545
xxx Bestwick, J. P., & Wald, N. J. (2015). Antenatal screening for down’s syndrome, trisomy 18, and trisomy 13: Reporting a single screening result for all three. Journal of Medical Screening, 22(2), 100-105. https://doi.org/10.1177/0969141315575545
xxxi Bestwick, J. P., & Wald, N. J. (2015). Antenatal screening for down’s syndrome, trisomy 18, and trisomy 13: Reporting a single screening result for all three. Journal of Medical Screening, 22(2), 100-105. https://doi.org/10.1177/0969141315575545
xxxii Gil, M. M., et al. (2015). Analysis of cell-free DNA in maternal blood in screening for fetal aneuploidies: Updated meta-analysis. Ultrasound in Obstetrics & Gynecology, 45(3), 249-266. https://doi.org/10.1002/uog.14791
xxxiii Gil, M. M., et al. (2015). Analysis of cell-free DNA in maternal blood in screening for fetal aneuploidies: Updated meta-analysis. Ultrasound in Obstetrics & Gynecology, 45(3), 249-266. https://doi.org/10.1002/uog.14791
xxxiv Gil, M. M., et al. (2015). Analysis of cell-free DNA in maternal blood in screening for fetal aneuploidies: Updated meta-analysis. Ultrasound in Obstetrics & Gynecology, 45(3), 249-266. https://doi.org/10.1002/uog.14791
xxxv Gil, M. M., et al. (2015). Analysis of cell-free DNA in maternal blood in screening for fetal aneuploidies: Updated meta-analysis. Ultrasound in Obstetrics & Gynecology, 45(3), 249-266. https://doi.org/10.1002/uog.14791
xxxvi Carlson, L. M., & Vore, N. L. (2017). Prenatal diagnosis: Screening and diagnostic tools. Obstetrics and Gynecology Clinics of North America, 44(2), 245-256. https://doi.org/10.1016/j.ogc.2017.02.004
xxxvii Rose, N. C., et al. (2020). Screening for fetal chromosomal abnormalities. Obstetrics and Gynecology, 136(4), e48-e69. https://doi.org/10.1097/AOG.0000000000004084
xxxviii Practice bulletin No. 162. (2016). Obstetrics & Gynecology, 127(5), e108-e122. https://doi.org/10.1097/aog.0000000000001405
xxxix Wapner, R. J., et al. (2012). Chromosomal microarray versus karyotyping for prenatal diagnosis. The New England Journal of Medicine, 367, 2175-2184. https://www.doi.org/10.1056/NEJMoa1203382
xl Practice bulletin No. 162. (2016). Obstetrics & Gynecology, 127(5), e108-e122. https://doi.org/10.1097/aog.0000000000001405
xli Practice bulletin No. 162. (2016). Obstetrics & Gynecology, 127(5), e108-e122. https://doi.org/10.1097/aog.0000000000001405
xlii Jackson, L. G., et al. (1992). A randomized comparison of transcervical and transabdominal chorionic-villus sampling. The New England Journal of Medicine, 327, 594-598. https://doi.org/10.1056/NEJM199208273270903
xliii Practice bulletin No. 162. (2016). Obstetrics & Gynecology, 127(5), e108-e122. https://doi.org/10.1097/aog.0000000000001405
xliv Practice bulletin No. 162. (2016). Obstetrics & Gynecology, 127(5), e108-e122. https://doi.org/10.1097/aog.0000000000001405
xlv Akolekar, R., et al. (2015). Procedure-related risk of miscarriage following amniocentesis and chorionic villus sampling. Obstetrical & Gynecological Survey, 70(6), 369-371. https://doi.org/10.1097/ogx.0000000000000214
xlvi Botto, L. D., et al. (1996). Chorionic villus sampling and transverse digital deficiencies: Evidence for anatomic and gestational-age specificity of the digital deficiencies in two studies. American Journal of Medical Genetics, 62(2), 173-178. https://doi.org/10.1002/(sici)1096-8628(19960315)62:2<173::aid-ajmg11>3.0.co;2-l
xlvii Practice bulletin No. 162. (2016). Obstetrics & Gynecology, 127(5), e108-e122. https://doi.org/10.1097/aog.0000000000001405
xlviii Practice bulletin No. 162. (2016). Obstetrics & Gynecology, 127(5), e108-e122. https://doi.org/10.1097/aog.0000000000001405
xlix Practice bulletin No. 162. (2016). Obstetrics & Gynecology, 127(5), e108-e122. https://doi.org/10.1097/aog.0000000000001405
l Practice bulletin No. 162. (2016). Obstetrics & Gynecology, 127(5), e108-e122. https://doi.org/10.1097/aog.0000000000001405
li Akolekar, R., et al. (2015). Procedure-related risk of miscarriage following amniocentesis and chorionic villus sampling. Obstetrical & Gynecological Survey, 70(6), 369-371. https://doi.org/10.1097/ogx.0000000000000214
lii Randomised trial to assess safety and fetal outcome of early and midtrimester amniocentesis. The Canadian Early and Mid-trimester Amniocentesis Trial (CEMAT) Group. (1998). Lancet (London, England), 351(9098), 242–247.
liii Practice bulletin No. 162. (2016). Obstetrics & Gynecology, 127(5), e108-e122. https://doi.org/10.1097/aog.0000000000001405
liv Akolekar, R., et al. (2015). Procedure-related risk of miscarriage following amniocentesis and chorionic villus sampling. Obstetrical & Gynecological Survey, 70(6), 369-371. https://doi.org/10.1097/ogx.0000000000000214
lv Akolekar, R., et al. (2015). Procedure-related risk of miscarriage following amniocentesis and chorionic villus sampling. Obstetrical & Gynecological Survey, 70(6), 369-371. https://doi.org/10.1097/ogx.0000000000000214
lvi Centers for Disease Control and Prevention. (2021). Facts about Down syndrome. https://www.cdc.gov/ncbddd/birthdefects/downsyndrome.html
lvii Cereda, A., & Carey, J. C. (2012). The trisomy 18 syndrome. Orphanet Journal of Rare Diseases, 7(1), 81. https://doi.org/10.1186/1750-1172-7-81
lviii Genetic and Rare Diseases Information Center (GARD). (2020). Trisomy 13. https://rarediseases.info.nih.gov/diseases/7341/trisomy-13
lix NORD (National Organization for Rare Disorders). (2019). Turner syndrome. https://rarediseases.org/rare-diseases/turner-syndrome/
lx Genetic and Rare Diseases Information Center. (2018). Klinefelter syndrome. https://rarediseases.info.nih.gov/diseases/8705/klinefelter-syndrome
lxi Wigby, K., et al. (2016). Expanding the phenotype of triple X syndrome: A comparison of prenatal versus postnatal diagnosis. American Journal of Medical Genetics Part A, 170(11), 2870-2881. https://doi.org/10.1002/ajmg.a.37688