What is mitochondrial replacement therapy?
Mitochondrial replacement therapy (MRT) is a novel technique that can be used alongside . During the process, mitochondrial DNA (mtDNA) that is inherited from the mother (via her oocyte/egg) is replaced by the mtDNA from a female donor.i Mitochondrial DNA is different from the DNA in the nucleus that codes most of the genetics of the embryo (discussed below).
MRT may be recommended if there are abnormalities in the intended mother’s mtDNA that can lead to devastating mitochondrial diseases in the baby. These diseases are passed through the mtDNA from the egg to the fetus and are associated with an array of health disorders that can lead to early death in children.ii
Babies conceived with the assistance of MRT contain nuclear DNA from the female providing the egg, nuclear DNA from the male providing the sperm, and mtDNA from the MRT donor. Though a baby created using MRT will contain DNA from three individuals, only a small portion of the baby’s total DNA comes from a mitochondrial donor.
What are mitochondria?
Mitochondria are small structures inside human cells that make most of the energy required for a cell to function. Using a process called cell respiration, the mitochondria produce small molecules called adenosine triphosphate (ATP), which is the main energy “currency” of the cell. It is used for everything a body’s cells do — from allowing muscles to contract to helping send nerve impulses. ATP is also essential for DNA replications.
Mitochondria are special for a variety of reasons. Perhaps most interestingly, they have their own DNA (mtDNA), which is genetic material separate from the primary DNA of the cell. The number of mitochondria in each human cell depends on how much energy is required for the function of that cell. For example, there are thousands of mitochondria in a human heart cell, making up 40 percent of the total cell mass, because huge amounts of energy are required by heart cells.iii For comparison, mitochondria comprise only 2 to 6 percent of the total volume of endothelial cells (the cells that line our blood vessels), as these cells do not require as much energy to function.
Oocytes are rich in mitochondria, with primary oocytes containing approximately 6,000 mitochondria per cell.iv Mature oocytes can have up to 100,000 mitochondria per cell.v

Mitochondrial DNA vs. nuclear DNA
To better understand mitochondrial replacement therapy and how it is performed, it is important to be familiar with the difference between mitochondrial DNA (mtDNA) and nuclear DNA (nDNA, also called genomic DNA). They have different locations and functions and are distributed differently when an embryo is formed after conception. Mitochondrial DNA is the type involved in the development of mitochondrial disease.
Mitochondria — and therefore mtDNA — are typically passed down exclusively from the egg to the embryo. Nuclear DNA, on the other hand, is passed down equally from the egg and the sperm (50 percent from the egg, 50 percent from the sperm).vi It is thought that when the sperm fertilizes the egg, the mitochondria inside the sperm head are destroyed. Almost always, only the egg mitochondria remain — with the DNA they contain— to become part of the embryo.vii
Mitochondrial DNA makes up only a small fraction of the total genetic material in a human cell. Current estimates are that there are approximately 21,000 genes in the nuclear human genome; mtDNA includes the sequences for only 37 genes. These 37 genes determine mitochondrial function.viii,ix
As each human cell has many mitochondria, there are many copies of mtDNA in each cell. In almost all cases, all of these mtDNA copies are identical (called homoplasmy) and the same as the mtDNA from the egg. In rare cases, mtDNA copies can be different (called heteroplasmy) due to a spontaneous mutation or a small amount of mtDNA inherited from the sperm.x Despite the small number of mtDNA genes, many of these genes encode proteins that are essential for egg and embryo survival.
Mutated mtDNA causes a series of genetic disorders known as mitochondrial diseases. Mitochondrial DNA mutations are detected in approximately 1 in 250 live births, and at least 1 in 10,000 adults are affected by a mitochondrial disease.xi,xii,xiii There are limited treatment options for mitochondrial diseases, and many of these disorders are fatal.xiv
Because of the inheritance pattern of mitochondria, inherited mitochondrial diseases are generally inherited from the mother, not the father. In some cases, a female using her own eggs to conceive is a carrier of the disease and does not have any symptoms, but all of her offspring will inherit the disease. Preimplantation genetic testing (PGT, which is genetic testing prior to embryo transfer) is not useful for patients with heteroplasmic mtDNA mutations, and not all genetic platforms offer testing for mitochondrial disease. The reason is that PGT checks primarily for abnormalities in nuclear DNA, not mitochondrial DNA.xv
There are dozens of mitochondrial diseases, many of which have severe symptoms or effects. These diseases are often disorders of the brain and/or muscles, with a range of symptoms such as epilepsy, blindness, muscle weakness, difficulty with balance, and respiratory failure. The exact symptoms vary by the type of mutation in the mtDNA.xvi,xvii The most common mitochondrial disease is Leber hereditary optic neuropathy (LHON), which causes permanent blindness before 40 years of age. While always inherited maternally, people who are carriers of the disease do not always have symptoms of the disease.xviii
The idea that MRT could address these serious genetic disorders is one reason why MRT has been an area of scientific study. If mitochondrial mutations are removed and replaced, the transmission of these devastating diseases may be prevented.
How does mitochondrial replacement therapy work?
In MRT, nuclear DNA from the donor oocyte (egg) is removed, leaving only the donor mitochondrial DNA and the rest of the donor oocyte contents. Then, the intended mother’s nuclear DNA is removed from her oocyte and transferred into the donor oocyte. Thus, the resulting oocyte will have 100 percent of its nDNA from the intended mother and 100 percent of its mtDNA from the donor.xxii This new oocyte can then be fertilized by sperm to form an embryo.
The egg and embryo structure
To better understand mitochondrial replacement therapy, one must first look at the egg and embryo structure, as well as how chromosomes are distributed. In primary human oocytes, the nuclear DNA is arranged inside the nucleus in pairs of 23 chromosomes, each with a set of chromatids (for a total of 46 chromosomes, called a diploid cell). As an oocyte matures, a process called meiosis divides up the nuclear DNA so that only half of the chromosome set is remaining (for a total of 23 chromosomes, called a haploid cell). There are two division steps in meiosis, and both are important in making sure that the final oocyte is genetically ready for fertilization by sperm. The combination of a haploid oocyte (23 chromosomes) and haploid sperm (23 chromosomes) leads to a diploid embryo (46 chromosomes).
During the first meiotic cell division, the envelope that keeps the nucleus separate from the rest of the cell dissolves, thus freeing the DNA to enter the cell cytoplasm. The DNA is lined up on a spindle apparatus made up of microtubules. This spindle apparatus is like strings or a web that orients each of the chromosomes in a line, so the chromosomes are evenly divided between newly formed cells as the oocyte divides.
The two cells that result from the first meiotic division are the secondary oocyte and the first polar body. Each of these resulting cells has 23 chromosomes, each with two chromatids. The first polar body contains less cytoplasm (and therefore much less mitochondria) than the secondary oocyte.
The secondary oocyte then undergoes a second meiotic division. As with the first meiotic division, the chromosomes are lined up by the spindle apparatus so that equal division of the chromosomes can occur. The secondary oocyte expels a second polar body with one set of chromatids, and now contains 23 chromosomes, each with one chromatid.
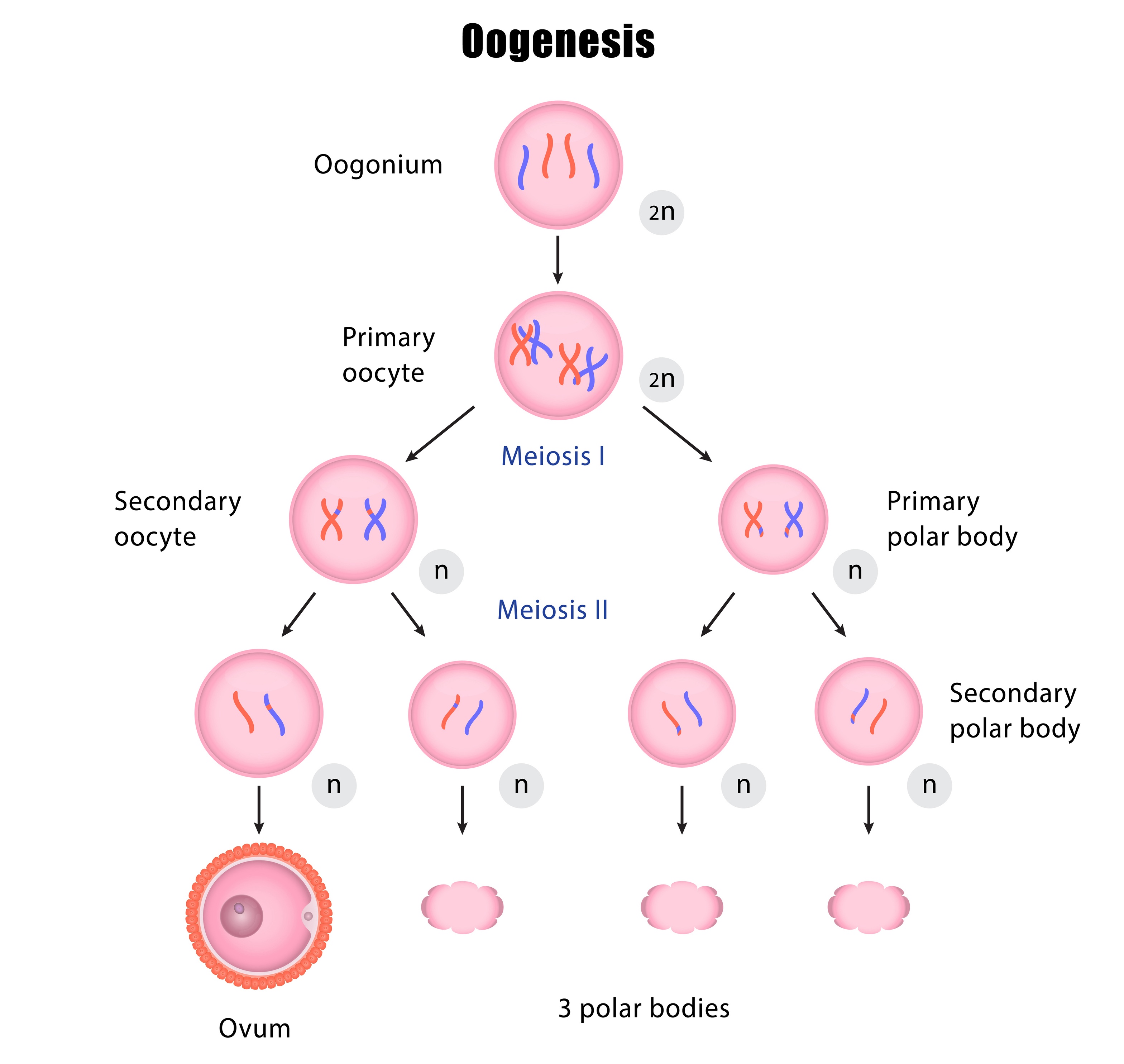
There are three types of mitochondrial replacement therapy:
- Maternal spindle transfer (MST)
- Pronuclear transfer (PNT)
- Polar body genome transfer (PBT), which is less common
Below is a further explanation of each type of MRT.
Maternal spindle transfer (MST)
Maternal spindle transfer (MST) involves transferring specific genetic material from the intended mother’s oocyte to the oocyte of the donor. This process takes place before the oocyte is fertilized to become an embryo.
Here is what happens during the MST technique:xxiii
- The intended mother’s chromosomes, which contain DNA arranged on spindles, are removed from one of her oocytes.
- This oocyte is then discarded.
- The nuclear chromosomes and spindle apparatus are removed from a donor oocyte and discarded.xxiv
- The intended mother’s nuclear chromosomes (suspended in the spindle apparatus) are then placed into the donor oocyte, which still contains all the donor mitochondria and their presumably healthy mitochondrial DNA.
In effect, the donor oocyte still contains all its original components, except the nuclear DNA has been replaced.xxv
The transfer of material will be repeated using more donor oocytes and nuclear DNA spindles from the mother. Once all the DNA spindles available from the intended mother have been transferred to all the donor oocytes, the oocytes will be fertilized in the lab. The embryos created will be grown in the lab until Day 3 or 5, after which an embryo transfer will be performed. Alternatively, embryos may be cryopreserved (frozen) for future use.
Pronuclear transfer (PNT)
In pronuclear transfer (PNT), these are the primary steps:xxvi
- Both the intended mother’s oocyte and the donor oocyte are fertilized by sperm. Upon successful fertilization, there are two pronuclei (2PN) within each embryo — one representing genetic material from the oocyte and one from the sperm.
- The two pronuclei in the embryo made from a donor oocyte (containing nDNA from the donor and the sperm) are removed from that embryo.
- The two pronuclei in the embryo made by the intended parents’ egg and sperm are removed from that embryo and placed into the donor-derived embryo (from which the 2PN had previously been removed).
- The empty embryo created from the intended mother's oocyte is then discarded, as it presumably contains mitochondrial mutations.
The reconstituted embryo will have nuclear DNA from the intended parents and presumably normal mtDNA from the donor.xxvii Embryo culture is performed, as in MST, followed by embryo transfer or cryopreservation.
Polar body genome transfer (PBT)
When oocytes divide, the division is typically asymmetric. Division creates a secondary oocyte (which will continue to mature) and another cell, which is called a polar body. The polar body is smaller, contains less cytoplasm, and will eventually degenerate 17 to 24 hours after being expelled from the oocyte.xxviii
Because polar bodies contain less cytoplasm, they contain very few mitochondria, and therefore, very little mitochondrial DNA. However, the polar body contains the same-sized nucleus and the same amount of nDNA as a secondary oocyte.xxix
In PBT, either 1) the entire polar body 1 of the intended mother is transferred into a donor oocyte (PB1T), which is then fertilized by sperm, or 2) both the donor oocyte and the intended mother’s polar body are fertilized by sperm to create two embryos. The pronucleus is removed from the donor-derived embryo so there is no nuclear genetic material in it, and the fertilized polar body from the intended mother is inserted inside the donor-derived embryo, which contains only mtDNA from the oocyte donor (PB2T).xxx
What are the pros and cons of mitochondrial replacement therapy?
There are both advantages and disadvantages to mitochondrial replacement therapy (MRT):
Pros
The primary advantage of MRT is the potential that a parent with mitochondrial disease could have offspring that are genetically related to them without the risks of mitochondrial disease transmission.xxxi,xxxii
Another advantage of MRT is that it allows the intended parent to be genetically related to their child, because genetic material is manipulated. Generally, when using an egg donor, the intended parent is not genetically related to the conceived child, since the nuclear genome of the donor is passed on to the offspring, not the intended parent’s nuclear genome.
Cons
It is unclear what, if any, possible genetic damage occurs when genetic material is extracted from an oocyte or early embryo and inserted into another oocyte or embryo. Manipulating the embryos in this manner could lead to abnormal embryo development.
One additional theoretical risk is that, since the mitochondrial DNA in an embryo created through MRT is from a different individual than the nuclear DNA, the mitochondria in the fetal cells may not interact normally with the nucleus of the cells. The possible effect of this interaction is unclear.xxxiii
Finally, MRT is an experimental therapy. The first live birth resulting from mitochondrial replacement therapy occurred in 2016, so there is limited data on success rates and currently no long-term follow-up documenting any possible future risks to children conceived with MRT.xxxiv
Due to the need for further research on the safety and efficacy of MRT, the current European Society of Human Reproduction and Embryology (ESHRE) guidelines state that this technique should only be used under strict research protocols that ensure the safety of the patients and provide long-term follow-up of any children born from MRT.xxxv In other words, ESHRE does not currently recommend MRT become commercially available at IVF clinics.
What is the history of mitochondrial replacement therapy?
MRT is not a well-established treatment option for fertility, so there is limited history of the procedure and its outcomes. This lack of data is largely because access to MRT remains limited to a few labs.
Successful MRT has been performed and is legal in Mexico, Ukraine, Greece, and Spain, and is legal in the United Kingdom as well. The first live birth of a boy conceived using the MST technique of mitochondrial replacement therapy was to a Jordanian couple in the U.S. in 2016, after treatment by a U.S.-based team in Mexico. The first live birth of a girl conceived using the PNT technique of mitochondrial replacement therapy was in Ukraine in 2017. There are reports of several additional live births in Ukraine, though the fertility group responsible has not published their results.xxxvi In 2023, there was a report of a baby born after mitochondrial donation therapy in the U.K., although no official reports have been published.xxxvii
The first pilot study of the effectiveness of the MST technique for patients with repeated IVF failures and no history of mitochondrial DNA diseases was conducted in Greece.xxxviii The study included 25 couples who underwent 28 IVF cycles. From these cycles, six babies were born and were developing normally by 12 to 24 months of age. Although this study provides insight into the feasibility of this technique, additional research is required to study whether it is effective at improving the chances of treatment success for patients with unexplained infertility.xxxix
What countries have access to mitochondrial replacement therapy?
MRT is extremely controversial due in part to the ethical questions surrounding the treatment. In the U.S., the FDA released a statement in 2018 affirming that MRT is currently not legal, even in a clinical trial or research setting.xl MRT is also not legally allowed in Canada or Israel and is restricted to research use in Australia.xli In Germany, laws are vaguer. There is no mention of MRT specifically in the German Embryo Protection Act, so MRT is technically legal, though no one is currently using this technology in Germany.xlii
The U.K. was the first country to legalize MRT, under the Human Fertilization and Embryology (Mitochondrial Donation) Regulations of 2015, though with some restrictions on the type of MRT and when and why it can be used. Presently, MRT is only legally permitted to prevent a mother from passing specific mitochondrial DNA diseases to her offspring; it cannot be used to treat infertility alone.xliii As mentioned above, MRT is also legal in Mexico, Ukraine, Greece, and Spain. However, that does not mean it is commercially available, or accessible.
What ethics questions surround mitochondrial replacement therapy?
While MRT may be a valuable tool in the prevention of genetic disease, objections to mitochondrial replacement techniques tend to stem from moral, social, or ethical concerns.
From a medical perspective, as MRT involves manipulating the genetic material of the resultant offspring, all future offspring of a female child born after MRT would have this same mitochondrial DNA. Thus, these genes would be passed on to future generations, as would any problem with the donated mtDNA.xliv
There are concerns from a social standpoint as well. The resultant child could struggle with their familial identity as they have genetic material from three individuals, which could raise confusing questions as to parentagexlv and result in legal issues. However, the U.K. addressed this concern through draft regulations explicitly stating that a mitochondrial donor would not be a legal parent to the child but more akin to an organ donor. Once again, it should be noted that, presently, MRT is only legally permitted in the U.K. to prevent a mother from passing a specific mitochondrial disease to her offspring. MRT cannot be used to treat infertility alone.xlvi
Moral objections have made MRT controversial in some countries. Many countries have restrictions on embryo research, which stems in part from religious concerns related to when life begins. This argument is often applied to MRT research.xlvii
Conclusion
Mitochondrial replacement therapy (MRT) may hold promise as an option in reproductive medicine, especially to help prevent genetic diseases. However, the procedure is highly controversial, with objections coming from ethical, moral, and social standpoints. Even though MRT is starting to gain acceptance as a therapeutic option in a few countries, it may be several years before the procedure is accepted on a broader scale.
Continued study of MRT may yield more insight into its effectiveness in helping prevent serious mitochondrial disease, its use in treating ovarian aging, and the long-term safety of the technology. For now, the option of mitochondrial donation and genetic engineering remains theoretical for most of the world.
i Sharma, H., et al. (2020). Development of mitochondrial replacement therapy: A review. Heliyon, 6(9), e04643. https://doi.org/10.1016/j.heliyon.2020.e04643
ii Sharma, H., et al. (2020). Development of mitochondrial replacement therapy: A review. Heliyon, 6(9), e04643. https://doi.org/10.1016/j.heliyon.2020.e04643
iii Li, A., et al. (2020). Mitochondrial dynamics in adult Cardiomyocytes and heart diseases. Frontiers in Cell and Developmental Biology, 8. https://doi.org/10.3389/fcell.2020.584800
iv Arbeithuber, B., et al. (2020). Age-related accumulation of de Novo mitochondrial mutations in mammalian oocytes and somatic tissues. PLOS Biology, 18(7), e3000745. https://doi.org/10.1371/journal.pbio.3000745
v Zhang, D., et al. (2017). Mitochondria in oocyte aging: current understanding. Facts, views & vision in ObGyn, 9(1), 29–38.
vi Luo, S., et al. (2018). Biparental inheritance of mitochondrial DNA in humans. Proceedings of the National Academy of Sciences, 115(51), 13039-13044. https://doi.org/10.1073/pnas.1810946115
vii Giles, R. E., et al. (1980). Maternal inheritance of human mitochondrial DNA. Proceedings of the National Academy of Sciences, 77(11), 6715-6719. https://doi.org/10.1073/pnas.77.11.6715
viii Willyard, C. (2018). New human gene tally reignites debate. Nature, 558(7710), 354-355. https://doi.org/10.1038/d41586-018-05462-w
ix Filograna, R., et al. (2020). Mitochondrial DNA copy number in human disease: The more the better? FEBS Letters, 595(8), 976-1002. https://doi.org/10.1002/1873-3468.14021
x Stewart, J. B., & Chinnery, P. F. (2015). The dynamics of mitochondrial DNA heteroplasmy: Implications for human health and disease. Nature Reviews Genetics, 16(9), 530-542. https://doi.org/10.1038/nrg3966
xi Craven, L., et al. (2010). Pronuclear transfer in human embryos to prevent transmission of mitochondrial DNA disease. Nature, 465(7294), 82-85. https://doi.org/10.1038/nature08958
xii Gorman, G. S., et al. (2015). Prevalence of nuclear and mitochondrial DNA mutations related to adult mitochondrial disease. Annals of Neurology, 77(5), 753-759. https://doi.org/10.1002/ana.24362
xiii Schaefer, A. M., et al. (2008). Prevalence of mitochondrial DNA disease in adults. Annals of Neurology, 63(1), 35-39. https://doi.org/10.1002/ana.21217
xiv Craven, L., et al. (2010). Pronuclear transfer in human embryos to prevent transmission of mitochondrial DNA disease. Nature, 465(7294), 82-85. https://doi.org/10.1038/nature08958
xv Smeets, H. J., et al. (2015). Preventing the transmission of mitochondrial DNA disorders using prenatal or preimplantation genetic diagnosis. Annals of the New York Academy of Sciences, 1350, 29–36. https://doi.org/10.1111/nyas.12866
xvi Chan, D. C. (2006). Mitochondria: Dynamic organelles in disease, aging, and development. Cell, 125(7), 1241-1252. https://doi.org/10.1016/j.cell.2006.06.010
xvii Bahr, T., et al. (2020). Emerging model systems and treatment approaches for Leber's hereditary optic neuropathy: Challenges and opportunities. Biochimica et Biophysica Acta (BBA) - Molecular Basis of Disease, 1866(6), 165743. https://doi.org/10.1016/j.bbadis.2020.165743
xviii Bahr, T., et al. (2020). Emerging model systems and treatment approaches for Leber's hereditary optic neuropathy: Challenges and opportunities. Biochimica et Biophysica Acta (BBA) - Molecular Basis of Disease, 1866(6), 165743. https://doi.org/10.1016/j.bbadis.2020.165743
xix National Academies of Sciences; Engineering; and Medicine, Institute of Medicine, Board on Health Sciences Policy, & Committee on the Ethical and Social Policy Considerations of Novel Techniques for Prevention of Maternal Transmission of Mitochondrial DNA Diseases. (2016). Mitochondrial replacement techniques: Ethical, social, and policy considerations. National Academies Press.
xx Wolf, D. P., et al. (2015). Mitochondrial replacement therapy in reproductive medicine. Trends in Molecular Medicine, 21(2), 68-76. https://doi.org/10.1016/j.molmed.2014.12.001
xxi Rose, N. C., et al. (2020). Screening for fetal chromosomal abnormalities. Obstetrics & Gynecology, 136(4), e48-e69. https://doi.org/10.1097/AOG.0000000000004084
xxii National Academies of Sciences; Engineering; and Medicine, Institute of Medicine, Board on Health Sciences Policy, & Committee on the Ethical and Social Policy Considerations of Novel Techniques for Prevention of Maternal Transmission of Mitochondrial DNA Diseases. (2016). Mitochondrial replacement techniques: Ethical, social, and policy considerations. National Academies Press.
xxiii National Academies of Sciences; Engineering; and Medicine, Institute of Medicine, Board on Health Sciences Policy, & Committee on the Ethical and Social Policy Considerations of Novel Techniques for Prevention of Maternal Transmission of Mitochondrial DNA Diseases. (2016). Mitochondrial replacement techniques: Ethical, social, and policy considerations. National Academies Press.
xxiv National Academies of Sciences; Engineering; and Medicine, Institute of Medicine, Board on Health Sciences Policy, & Committee on the Ethical and Social Policy Considerations of Novel Techniques for Prevention of Maternal Transmission of Mitochondrial DNA Diseases. (2016). Mitochondrial replacement techniques: Ethical, social, and policy considerations. National Academies Press.
xxv National Academies of Sciences; Engineering; and Medicine, Institute of Medicine, Board on Health Sciences Policy, & Committee on the Ethical and Social Policy Considerations of Novel Techniques for Prevention of Maternal Transmission of Mitochondrial DNA Diseases. (2016). Mitochondrial replacement techniques: Ethical, social, and policy considerations. National Academies Press.
xxvi National Academies of Sciences; Engineering; and Medicine, Institute of Medicine, Board on Health Sciences Policy, & Committee on the Ethical and Social Policy Considerations of Novel Techniques for Prevention of Maternal Transmission of Mitochondrial DNA Diseases. (2016). Mitochondrial replacement techniques: Ethical, social, and policy considerations. National Academies Press.
xxvii National Academies of Sciences; Engineering; and Medicine, Institute of Medicine, Board on Health Sciences Policy, & Committee on the Ethical and Social Policy Considerations of Novel Techniques for Prevention of Maternal Transmission of Mitochondrial DNA Diseases. (2016). Mitochondrial replacement techniques: Ethical, social, and policy considerations. National Academies Press.
xxviii Schmerler, S., & Wessel, G. M. (2010). Polar bodies-more a lack of understanding than a lack of respect. Molecular Reproduction and Development, 78(1), 3-8. https://doi.org/10.1002/mrd.21266
xxix National Academies of Sciences; Engineering; and Medicine, Institute of Medicine, Board on Health Sciences Policy, & Committee on the Ethical and Social Policy Considerations of Novel Techniques for Prevention of Maternal Transmission of Mitochondrial DNA Diseases. (2016). Mitochondrial replacement techniques: Ethical, social, and policy considerations. National Academies Press.
xxx National Academies of Sciences; Engineering; and Medicine, Institute of Medicine, Board on Health Sciences Policy, & Committee on the Ethical and Social Policy Considerations of Novel Techniques for Prevention of Maternal Transmission of Mitochondrial DNA Diseases. (2016). Mitochondrial replacement techniques: Ethical, social, and policy considerations. National Academies Press.
xxxi Tachibana, M., et al. (2018). Mitochondrial replacement therapy and assisted reproductive technology: A paradigm shift toward treatment of genetic diseases in gametes or in early embryos. Reproductive Medicine and Biology, 17(4), 421-433. https://doi.org/10.1002/rmb2.12230
xxxii Rulli, T. (2016). What is the value of three-parent IVF? Hastings Center Report, 46(4), 38-47. https://doi.org/10.1002/hast.594
xxxiii Farnezi, H. C., et al. (2020). Three-parent babies: Mitochondrial replacement therapies. JBRA Assisted Reproduction. https://doi.org/10.5935/1518-0557.20190086
xxxiv Zhang, J., et al. (2017). Live birth derived from oocyte spindle transfer to prevent mitochondrial disease. Obstetrical & Gynecological Survey, 72(9), 542-543. https://doi.org/10.1097/01.ogx.0000524508.40309.08
xxxv Lundin, K., et al. (2023). Good practice recommendations on add-ons in reproductive medicine. Human Reproduction, 38(11), 2062-2104. https://doi.org/10.1093/humrep/dead184
xxxvi Cohen, I. G., et al. (2020). The regulation of mitochondrial replacement techniques around the world. Annual Review of Genomics and Human Genetics, 21(1), 565-586. https://doi.org/10.1146/annurev-genom-111119-101815
xxxvii Wise, J. (2023). First baby born in the UK using mitochondrial donation therapy. BMJ, p1091. https://doi.org/10.1136/bmj.p1091
xxxviii Costa-Borges, N., et al. (2023). First pilot study of maternal spindle transfer for the treatment of repeated in vitro fertilization failures in couples with idiopathic infertility. Fertility and Sterility, 119(6), 964-973. https://doi.org/10.1016/j.fertnstert.2023.02.008
xxxix Costa-Borges, N., et al. (2023). First pilot study of maternal spindle transfer for the treatment of repeated in vitro fertilization failures in couples with idiopathic infertility. Fertility and Sterility, 119(6), 964-973. https://doi.org/10.1016/j.fertnstert.2023.02.008
xl U.S. Food and Drug Administration. (2018). Advisory on legal restrictions on the use of mitochondrial replacement. https://www.fda.gov/vaccines-blood-biologics/cellular-gene-therapy-products/advisory-legal-restrictions-use-mitochondrial-replacement-techniques-introduce-donor-mitochondria
xli Cohen, I. G., et al. (2020). The regulation of mitochondrial replacement techniques around the world. Annual Review of Genomics and Human Genetics, 21(1), 565-586. https://doi.org/10.1146/annurev-genom-111119-101815
xlii Cohen, I. G., et al. (2020). The regulation of mitochondrial replacement techniques around the world. Annual Review of Genomics and Human Genetics, 21(1), 565-586. https://doi.org/10.1146/annurev-genom-111119-101815
xliii Cohen, I. G., et al. (2020). The regulation of mitochondrial replacement techniques around the world. Annual Review of Genomics and Human Genetics, 21(1), 565-586. https://doi.org/10.1146/annurev-genom-111119-101815
xliv Craven, L., Murphy, J., Turnbull, D. M., Taylor, R. W., Gorman, G. S., & McFarland, R. (2018). Scientific and ethical issues in mitochondrial donation. The New Bioethics, 24(1), 57-73. https://doi.org/10.1080/20502877.2018.
xlv Craven, L., Murphy, J., Turnbull, D. M., Taylor, R. W., Gorman, G. S., & McFarland, R. (2018). Scientific and ethical issues in mitochondrial donation. The New Bioethics, 24(1), 57-73. https://doi.org/10.1080/20502877.2018.1440725
xlvi Klitzman, R., et al. (2015). Controversies concerning mitochondrial replacement therapy. Fertility and Sterility, 103(2), 344-346. https://doi.org/10.1016/j.fertnstert.2014.10.028
xlvii Craven, L., Murphy, J., Turnbull, D. M., Taylor, R. W., Gorman, G. S., & McFarland, R. (2018). Scientific and ethical issues in mitochondrial donation. The New Bioethics, 24(1), 57-73. https://doi.org/10.1080/20502877.2018.1440725