What is a follicle?
An ovarian follicle is a small fluid-filled sac that contains one egg or oocyte. At birth, each ovary contains hundreds of thousands of immature follicles, known as primordial follicles.i An individual will not create any more follicles (or eggs) during her lifetime. The collection of primordial follicles is the total supply of follicles and eggs that will be available for reproduction during a person’s lifetime. What is important within the context of fertility is the number of oocytes present at birth and their rate of decline.ii
Primordial follicles are in a dormant or resting phase at birth. They are simple, small structures (≈15 micrometers [μm] to 40μm in diameter) and consist of a single layer of flat support cells surrounding a single primary oocyte.iii
Primordial follicles can evolve in four different ways:iv
- Remain in their dormant state for the entire human lifespan
- Undergo apoptosis (die off)
- Begin to develop and then undergo apoptosis
- Begin development and progress to ovulation, where the oocyte is released from the follicle
The oocyte inside a primordial follicle is called a primary oocyte. These oocytes are paused in the prophase part of meiosis I. This is the beginning of the series of steps called meiosis, which is the process of cell division to create mature oocytes for reproduction. These immature oocytes are referred to as germinal vesicle (GV) oocytes. Primary oocytes are not yet mature and cannot be fertilized.v
All follicles stay dormant in the primordial follicle state until puberty when menstruation starts.viAt the beginning of each menstrual cycle, a number of primordial follicles are triggered to begin development toward becoming primary follicles. Like primordial follicles, primary follicles have a single layer of support cells (granulosa cells) surrounding a central primary oocyte.vii However, the follicle grows in size (roughly 100 μm in diameter) and the granulosa cells become square in shape (cuboidal).
Primary follicles also develop a zona pellucida, a thin shell made of glycoproteins (carbohydrates linked to proteins) that surrounds the oocyte. Development of this shell is essential for normal fertilization.viii The primary oocyte inside the primary follicle starts to grow dramatically, and primary follicles develop receptors to follicle stimulating hormone (FSH), which allow the follicle and oocyte to continue developing in response to FSH.ix
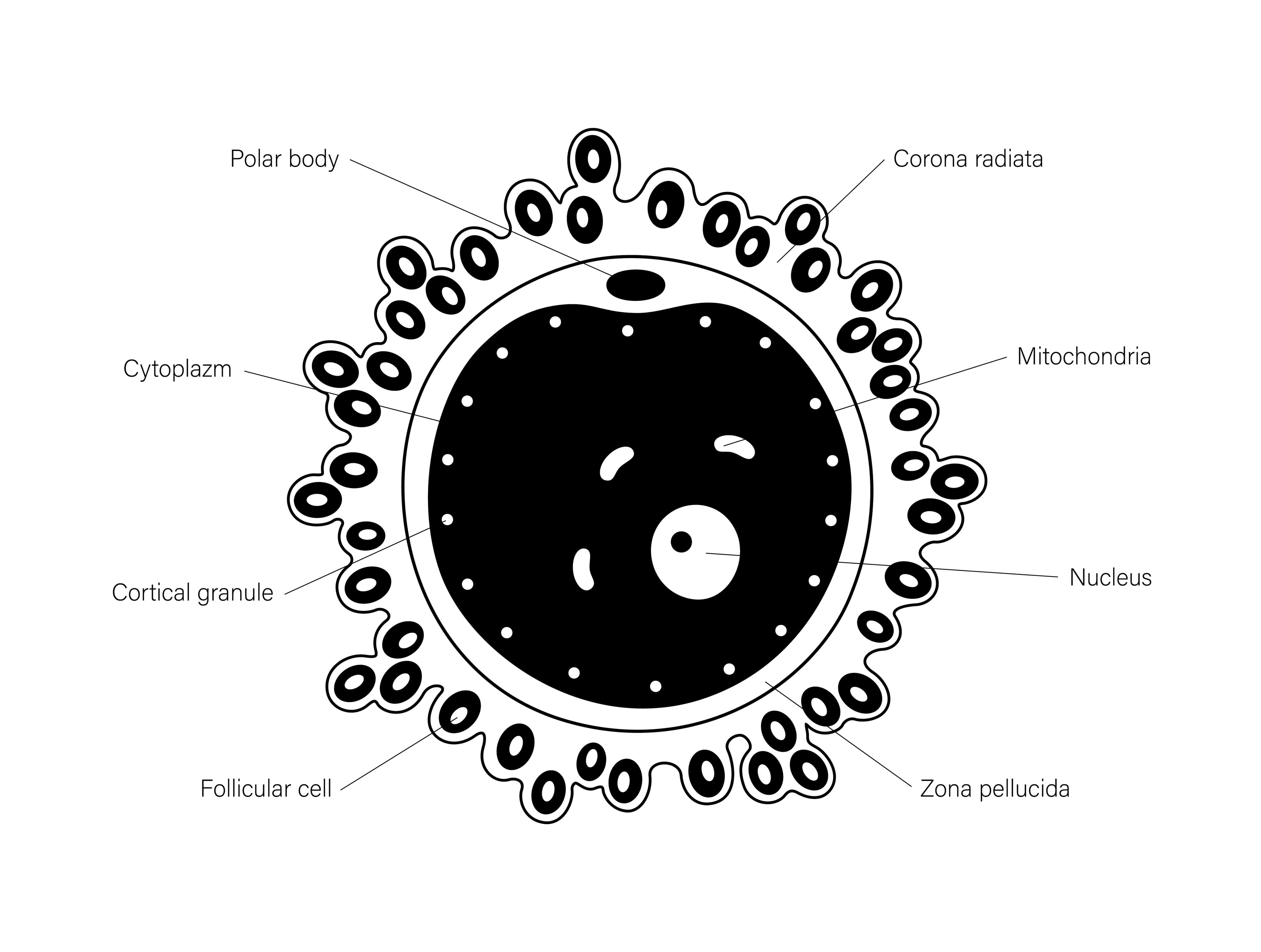
Some primary follicles develop into secondary follicles, which are larger (on average 200 μm), contain a more developed oocytex and have more granulosa.xi Those layers protect the oocyte and aid in follicle development through exposure to hormonal signaling from FSH, luteinizing hormone (LH), and insulin.xii
During a process called cavitation, secondary follicles develop a fluid cavity called an antrum.xiii At this point, the follicle is called an antral, tertiary, or Graafian follicle. FSH and LH signaling controls this process. In the tertiary stage, the oocyte in the follicle is fully developed, measuring around 120 μm.xiv The follicle will continue to increase in size, up to 2cm, but the oocyte will not.xv One of these antral follicles becomes the dominant follicle and will continue to grow during the menstrual cycle. The rest of the follicles (primary, secondary and antral) undergo apoptosis (programmed cell death).xvi
It takes more than 300 days for a primordial follicle to be selected into the follicular pool as a primary oocyte and reach the final phase of development as an antral follicle before ovulation. At any given point during the menstrual cycle, there are follicles at all stages of development.xvii
In a typical menstrual cycle, the follicle reaches its maximum size and maturity and is ready to ovulate around day 12-16. The pituitary gland then releases LH (LH surge), causing final maturation of the oocyte and release of the oocyte into the pelvis to be picked up by the tube. The oocyte resumes meiosis following the LH surge, becoming a secondary oocyte or MII (metaphase of meiosis II) oocyte, which is now capable of being fertilized.xviii After ovulation, the follicle from which the oocyte was released becomes a corpus luteum cyst, xix producing progesterone and estradiol (an estrogen derivative) to support implantation if fertilization occurs and an embryo is formed.xx
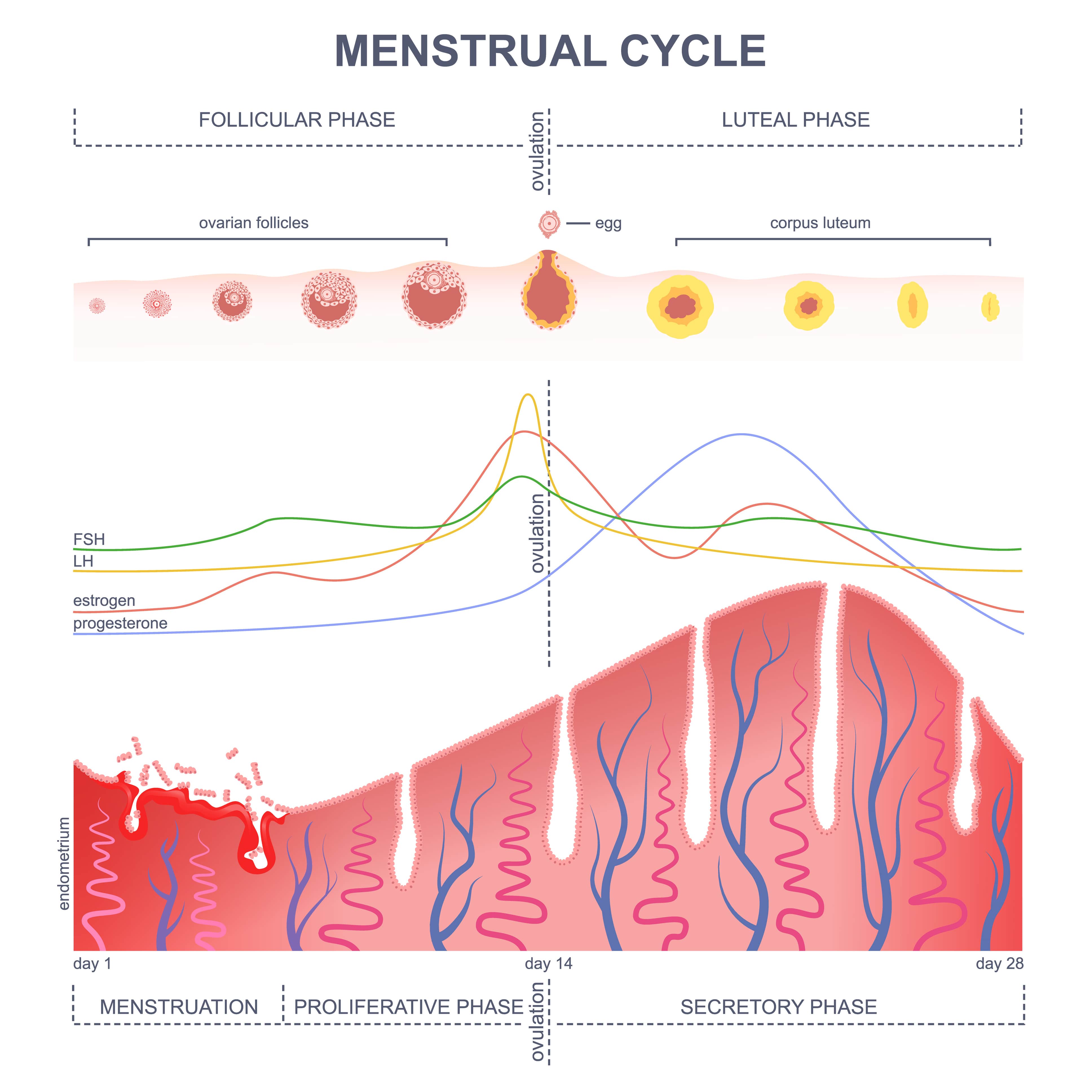
If an embryo does not implant, the corpus luteum undergoes apoptosis and there is a drop in progesterone levels, leading to menses.xxi If an embryo implants in the uterus, b-hCG hormone is produced, which maintains the corpus luteum, ensuring continued production of progesterone and estradiol to support the early pregnancy.xxii
What are cysts?
Follicles are sometimes referred to as cysts, or functional cysts, which are normal follicles developing each month during a menstrual cycle.xxiii The term cyst is used clinically to describe a fluid-filled sac structure. This can be confusing for patients since often people think of cysts as abnormal or pathologic.
Follicular cysts from a previous cycle are not uncommon and occur when follicles fail to resolve and fill with fluid. These appear as simple cysts measuring 1-4 cm on ultrasound and usually resolve on their own in 4-6 weeks. The exact frequency of these cysts is not known since the majority cause no symptoms and go unnoticed. They are usually discovered incidentally on examination for another reason, such as on ultrasound during fertility treatment.xxiv In rarer cases, the cyst can be larger or cause pelvic pain and should be assessed by a physician.
Hemorrhagic cysts are blood-filled cysts, usually from a follicle that ovulated an oocyte in a previous cycle. These will also usually resolve on their own in 4-6 weeks. Hemorrhagic cysts are different than endometriomas, which are cysts filled with old blood that are associated with endometriosis and do not resolve on their own. These two types of blood-filled cysts usually look different on ultrasound.xxv
Other benign cysts include cystadenomas, cystadenofibromas, and dermoid cysts. These each have characteristic findings on ultrasound and do not originate from follicles in a previous cycle. Cysts can also be pre-cancerous (borderline tumors) or ovarian cancer. It is important to discuss with your physician the type of cyst noted on imaging and any recommended follow-up.
What is an egg (oocyte)?
An oocyte is the female reproductive cell, or gamete, located in the ovaries. Typically, women are born with 1-2 million primary oocytes. This drops to 300,000-500,000 oocytes at puberty and about 25,000 at age 37.xxvi Only around 400 oocytes will be ovulated from dominant follicles throughout the reproductive lifespan.xxvii That means 99.9 percent of follicles and oocytes will undergo apoptosis. Only 0.1 percent will mature to ovulation.xxviii
Every oocyte is contained in a follicle, as described above. Those eggs inside a primordial follicle are called primary oocytes. Primary oocytes are not mature and cannot be fertilized. Genetic material (DNA) in a primary oocyte is susceptible to damage over time. This sustained damage is one reason why the chromosomes in eggs may not always separate correctly, causing an abnormal chromosome number (aneuploidy) in embryos. As the oocytes age, reactive oxygen species (ROS) can also damage DNA and impair the egg’s ability to mature and function normally.xxix
As mentioned above, hormonal signaling from the brain (FSH and LH) triggers development of a dominant follicle. The LH surge causes final maturation of the oocyte, in which meiosis resumes and the oocyte progresses to an MII oocyte (metaphase of meiosis II). This maturation is required for normal fertilization.

During IVF, physicians stimulate growth of multiple dominant follicles to retrieve as many mature (MII) oocytes as possible. A trigger shot is given in IVF cycles to promote this final oocyte maturation, similar to the LH surge in a natural menstrual cycle. Even with a trigger shot, however, not all oocytes retrieved are in the MII stage; some will be immature.xxx Only mature oocytes can become fertilized and develop into an embryo.
How is ovarian reserve measured?
Ovarian reserve is the reproductive potential left in a woman’s two ovaries, based on the number of remaining follicles and eggs. Ovarian reserve naturally declines as a woman ages.
A number of factors influence ovarian reserve:
- Age
- Genetics
- Certain health conditions
- Environmental influences
Ovarian reserve decreases at different rates throughout a woman’s life. Oocyte depletion starts occurring at a faster rate when individuals reach their mid-30s.xxxi
Ovarian reserve can be measured in three ways.
Antimullerian Hormone (AMH) levels:
AMH is a glycoprotein (carbohydrate linked to a protein) made by the granulosa cells of the preantral and antral follicles. AMH can be measured in the blood with a laboratory test.xxxii,xxxiii
AMH begins to rise during puberty and typically peaks around age 25. It then declines until a few years prior to menopause.xxxiv Levels generally remain consistent during the menstrual cycle, so AMH can be measured on any cycle day.xxxv,xxxvi,xxxvii Hormonal medication, such as birth control pills and progesterone IUDs, can slightly lower the AMH level, so it is important for women to mention this to their doctor if they check AMH while on these medications.xxxviii
AMH correlates with the primordial follicle pool and predicts how well individuals will respond to ovarian stimulation with assisted reproductive technology (ART). A meta-analysis from 2013 found that lower AMH predicted poor outcomes (defined as <4 oocytes retrieved) with IVF.xxxix,xl,xli Normal AMH levels vary with age. The lower limit for normal AMH by age is:xlii,xliii,xliv
- 45yo: 0.5ng/mL
- 40yo: 1ng/mL
- 35yo: 1.5ng/mL
- 30yo: 2.5ng/mL
- 25yo: 3.0ng/mL
Overall, AMH of >1.7ng/mL is linked to good IVF outcomes and to acceptable ovarian reserve.xlv,xlvi Importantly, AMH is not a marker for fertility. Studies have shown equivalent fertility rates for people with low and normal AMH levels.xlvii,xlviii
Follicle Stimulating Hormone (FSH) levels:
Early follicular phase FSH level is a blood test that measures FSH in the bloodstream during days 2 to 4 of the menstrual cycle.xlix Ovaries in women with normal ovarian reserve make enough of the ovarian hormones estradiol and inhibin B to keep FSH low in the first few days of the menstrual cycle. FSH levels rise early in the cycle if the ovaries fail to produce enough estradiol and inhibin B, which occurs in the setting of diminished ovarian reserve.l
A normal early follicular phase FSH is typically <10 IU/L. When levels are consistently higher than that, this is a marker of lower ovarian reserve. It is important to also check estradiol level with the FSH level. If the estradiol level is high (>80-100 pg/mL), FSH will be artificially lowered. A high estradiol level early in the cycle is also a marker of low ovarian reserve. IVF patients with high early follicular FSH levels may not respond well to ovarian stimulation medication.li,lii
Antral Follicle Count (AFC):
This is an ultrasound measurement of ovarian reserve that is often used in addition to blood FSH and AMH measurement. The number of antral follicles helps predict the ovaries’ responsiveness to ovarian stimulation medication in IVF.
The test is typically conducted between day 2 and day 5 of the menstrual cycle. A transvaginal ultrasound is used to count the number of antral follicles, small fluid-filled cysts measuring 2 -10mm in each ovary.liii,liv
How many follicles are considered normal?
On average, women are born with 1-2 million primordial follicles, and each contains a primary oocyte.lv The number of ovarian follicles declines over time as follicles go through development cycles, and over 99 percent eventually undergo apoptosis (programmed cell death).
The number of antral follicles (follicles recruited for that specific menstrual cycle) can be measured on ultrasound by performing an antral follicle count (AFC). The definition of normal AFC varies based on the study referenced, and there is a large variation based on age. For example, females 20-34 years can generally expect a higher AFC (average 14-15) than those 38-46 years (average 4-5).lvi,lvii There is also a great deal of variation between individuals of the same age, and AFC can vary in the same individual from one cycle to the next. AFC also varies by diagnosis. Patients with polycystic ovary syndrome (PCOS) have much higher AFC than patients of the same age without PCOS.lviii
Although normal AFC cut-off values vary between studies, most providers agree that fewer than five total antral follicles is a sign of diminished ovarian reserve and predicts poor ovarian response to IVF medications.lix
It is important to note, however, that even if a woman has fewer than five antral follicles seen on ultrasound, that does not necessarily mean she will not respond to ovarian stimulation or not be able to conceive.lx
How many follicles and eggs are needed for IVF?
According to clinical studies, the average number of eggs retrieved during an IVF cycle varies from 7 to 9 eggs. This results in an average of 3 to 5 embryos.lxi The number of eggs retrieved is variable and depends on age and ovarian reserve. Studies show that, in general, the number of eggs retrieved correlates with age. It is important to note that not all retrieved eggs are mature, not all mature eggs will successfully fertilize to become an embryo, and not all embryos will continue to develop and be able to be used for transfer into the uterus.lxii
Not surprisingly, research shows that the more oocytes retrieved (up to around 15 oocytes), the higher the likelihood of pregnancy success.lxiii,lxiv Based on findings from several studies conducted between 2010 and 2014, retrieving between six and 15 eggs during a cycle is best to reach optimal pregnancy outcomes.lxv,lxvi,lxvii,lxviii,lxix These studies also showed a plateau effect beyond approximately 15 retrieved oocytes. The chance of a live birth increased only up to that number, and after that point, there was no improvement in live birth rate for that cycle.lxx,lxxi Of note, higher estradiol levels, a marker of egg development, on the day of ovulation trigger are associated with a higher number of oocytes retrieved.lxxii
How big should follicles be before triggering during fertility treatment?
Follicle size is constantly monitored during an IVF cycle. The size of the follicles on the day of trigger correlates with the number of oocytes that are successfully retrieved.lxxiii,lxxiv Transvaginal ultrasound is used to measure follicle size. Many researchers suggest waiting to trigger until at least two follicles are >16-18mm in size.lxxv
There is debate around the optimal size of follicles for ovulation trigger. Many studies have observed that follicles measuring less than 12 mm on the day of trigger are less likely to yield mature (MII) oocytes.lxxvi,lxxvii,lxxviii According to a 2017 Ultrasound in Obstetrics and Gynecology study, the authors measured follicle size at the time of 118 egg retrievals and found that follicle sizes from 13mm to 23mm had higher oocyte recovery rates and potential to yield good quality blastocysts.lxxix Similar results were found in a 2022 Fertility and Sterility study which looked at results from 157 oocyte retrievals.lxxx Regardless, it is recommended by some that even oocytes in small follicles present at the time of retrieval are collected (8mm-to-12mm), since eggs in these follicles may still lead to a live birth in some cases.lxxxi
Is it possible to have an empty follicle? What is empty follicle syndrome? How is it treated?
In a typical IVF cycle, the number of eggs retrieved can be lower than the number of follicles observed at the time of retrieval. That means an egg is not recovered from every emptied follicle.lxxxii The oocyte recovery rate refers to the percentage of aspirated follicles that result in a retrieved oocyte.lxxxiii Based on published literature, depending on size of follicles aspirated and aspiration technique, the average oocyte recovery rate ranges from 40 to 80 percent.lxxxiv In general, the larger the follicle, the more likely an oocyte will be aspirated from it.lxxxv
Empty follicle syndrome (EFS) is the extreme end of low oocyte recovery rate. It is rare, occurring in 0.2 to 7 percent of IVF cycles.lxxxvi With this condition, adequate stimulation is evident. Ovarian follicles appear normal, and estradiol production is appropriate. However, no oocytes are obtained from any successfully punctured and aspirated follicles.lxxxvii
Why EFS occurs is not exactly known. The most common theories suggest that aspiration fails to yield an oocyte because it has undergone apoptosis, the follicles are dysfunctional, or the oocytes failed to develop and mature normally. It is also possible that the trigger was not successful or premature ovulation occurred, meaning the oocyte was released prior to retrieval. Other reasons include genetically abnormal oocytes or abnormal hormonal receptors on the follicle.lxxxviii
For patients who have genuine EFS—correctly administered hCG trigger but no retrieved oocytes—few treatments are available to prevent it from happening in the future. However, there are some techniques that are worth investigating:lxxxix
- Increasing the time from hCG trigger to retrieval
- Giving a second hCG trigger treatment prior to retrieval
- Using dual hCG and GnRH agonist to trigger
Using these techniques, 86 percent of patients in one 2017 Reproductive BioMedicine Online study were able to obtain mature oocytes in a subsequent cycle.xc
Conclusion
The terminology and processes involved in IVF can be daunting to those who are new to the world of IVF treatment. In particular, the egg retrieval process and all that it entails are often difficult to grasp, which is not surprising given the precision of each measurement at each stage. Having a better understanding of the differences between follicles and eggs can provide clarity in terms of how each IVF cycle is progressing, thereby making it easier to set expectations during each step.
i Findlay, J., et al. (2019). Follicle selection in mammalian ovaries. The Ovary, 3-21. https://doi.org/10.1016/b978-0-12-813209-8.00001-7
ii Findlay, J., et al. (2019). Follicle selection in mammalian ovaries. The Ovary, 3-21. https://doi.org/10.1016/b978-0-12-813209-8.00001-7
iii Cordeiro, M. H., et al. (2015). Ovarian follicle biology and the basis for Gonadotoxicity. Cancer Treatment and the Ovary, 3-20. https://doi.org/10.1016/b978-0-12-801591-9.00001-1
iv Conti, M., & Chang, R. J. (2016). Folliculogenesis, ovulation, and Luteogenesis. Endocrinology: Adult and Pediatric, 2179-2191.e3. https://doi.org/10.1016/b978-0-323-18907-1.00125-6
v Conti, M., & Chang, R. J. (2016). Folliculogenesis, ovulation, and Luteogenesis. Endocrinology: Adult and Pediatric, 2179-2191.e3. https://doi.org/10.1016/b978-0-323-18907-1.00125-6
vi Cordeiro, M. H., et al. (2015). Ovarian follicle biology and the basis for Gonadotoxicity. Cancer Treatment and the Ovary, 3-20. https://doi.org/10.1016/b978-0-12-801591-9.00001-1
vii Histology at Yale. (n.d.). Ovary and follicle development. https://medcell.med.yale.edu/histology/ovary_follicle.php
viii Histology at Yale. (n.d.). Ovary and follicle development. https://medcell.med.yale.edu/histology/ovary_follicle.php
ix Conti, M., & Chang, R. J. (2016). Folliculogenesis, ovulation, and Luteogenesis. Endocrinology: Adult and Pediatric, 2179-2191.e3. https://doi.org/10.1016/b978-0-323-18907-1.00125-6
x Conti, M., & Chang, R. J. (2016). Folliculogenesis, ovulation, and Luteogenesis. Endocrinology: Adult and Pediatric, 2179-2191.e3. https://doi.org/10.1016/b978-0-323-18907-1.00125-6
xi Conti, M., & Chang, R. J. (2016). Folliculogenesis, ovulation, and Luteogenesis. Endocrinology: Adult and Pediatric, 2179-2191.e3. https://doi.org/10.1016/b978-0-323-18907-1.00125-6
xii Conti, M., & Chang, R. J. (2016). Folliculogenesis, ovulation, and Luteogenesis. Endocrinology: Adult and Pediatric, 2179-2191.e3. https://doi.org/10.1016/b978-0-323-18907-1.00125-6
xiii Conti, M., & Chang, R. J. (2016). Folliculogenesis, ovulation, and Luteogenesis. Endocrinology: Adult and Pediatric, 2179-2191.e3. https://doi.org/10.1016/b978-0-323-18907-1.00125-6
xiv Conti, M., & Chang, R. J. (2016). Folliculogenesis, ovulation, and Luteogenesis. Endocrinology: Adult and Pediatric, 2179-2191.e3. https://doi.org/10.1016/b978-0-323-18907-1.00125-6
xv Conti, M., & Chang, R. J. (2016). Folliculogenesis, ovulation, and Luteogenesis. Endocrinology: Adult and Pediatric, 2179-2191.e3. https://doi.org/10.1016/b978-0-323-18907-1.00125-6
xvi Conti, M., & Chang, R. J. (2016). Folliculogenesis, ovulation, and Luteogenesis. Endocrinology: Adult and Pediatric, 2179-2191.e3. https://doi.org/10.1016/b978-0-323-18907-1.00125-6
xvii Conti, M., & Chang, R. J. (2016). Folliculogenesis, ovulation, and Luteogenesis. Endocrinology: Adult and Pediatric, 2179-2191.e3. https://doi.org/10.1016/b978-0-323-18907-1.00125-6
xviii Conti, M., & Chang, R. J. (2016). Folliculogenesis, ovulation, and Luteogenesis. Endocrinology: Adult and Pediatric, 2179-2191.e3. https://doi.org/10.1016/b978-0-323-18907-1.00125-6
xix Conti, M., & Chang, R. J. (2016). Folliculogenesis, ovulation, and Luteogenesis. Endocrinology: Adult and Pediatric, 2179-2191.e3. https://doi.org/10.1016/b978-0-323-18907-1.00125-6
xx Conti, M., & Chang, R. J. (2016). Folliculogenesis, ovulation, and Luteogenesis. Endocrinology: Adult and Pediatric, 2179-2191.e3. https://doi.org/10.1016/b978-0-323-18907-1.00125-6
xxi Conti, M., & Chang, R. J. (2016). Folliculogenesis, ovulation, and Luteogenesis. Endocrinology: Adult and Pediatric, 2179-2191.e3. https://doi.org/10.1016/b978-0-323-18907-1.00125-6
xxii Conti, M., & Chang, R. J. (2016). Folliculogenesis, ovulation, and Luteogenesis. Endocrinology: Adult and Pediatric, 2179-2191.e3. https://doi.org/10.1016/b978-0-323-18907-1.00125-6
xxiii Mobeen, S., “Ovarian Cyst.“ National Center for Biotechnology Information (2021). https://www.ncbi.nlm.nih.gov/books/NBK560541/
xxiv Practice bulletin No. 174: Evaluation and management of Adnexal masses. (2016). Obstetrics & Gynecology, 128(5), e210-e226. https://doi.org/10.1097/aog.0000000000001768
xxv Levine, D., et al. (2010). Management of asymptomatic ovarian and other Adnexal cysts imaged at US: Society of radiologists in ultrasound consensus conference statement. Radiology, 256(3), 943-954. https://doi.org/10.1148/radiol.10100213
xxvi Female age-related fertility decline. (2014). Fertility and Sterility, 101(3), 633-634. https://doi.org/10.1016/j.fertnstert.2013.12.032
xxvii Conti, M., & Chang, R. J. (2016). Folliculogenesis, ovulation, and Luteogenesis. Endocrinology: Adult and Pediatric, 2179-2191.e3. https://doi.org/10.1016/b978-0-323-18907-1.00125-6
xxviii Conti, M., & Chang, R. J. (2016). Folliculogenesis, ovulation, and Luteogenesis. Endocrinology: Adult and Pediatric, 2179-2191.e3. https://doi.org/10.1016/b978-0-323-18907-1.00125-6
xxix Astbury, P., et al. (2020). The presence of immature GV− stage oocytes during IVF/ICSI is a marker of poor oocyte quality: A pilot study. Medical Sciences, 8(1), 4. https://doi.org/10.3390/medsci8010004
xxx Astbury, P., et al. (2020). The presence of immature GV− stage oocytes during IVF/ICSI is a marker of poor oocyte quality: A pilot study. Medical Sciences, 8(1), 4. https://doi.org/10.3390/medsci8010004
xxxi Tal, R., & Seifer, D. B. (2017). Ovarian reserve testing: A user’s guide. American Journal of Obstetrics and Gynecology, 217(2), 129-140. https://doi.org/10.1016/j.ajog.2017.02.027
xxxii La Marca, A., et al. (2009). Anti-mullerian hormone (AMH) as a predictive marker in assisted reproductive technology (ART). Human Reproduction Update, 16(2), 113-130. https://doi.org/10.1093/humupd/dmp036
xxxiii Seifer, D. B., et al. (1993). Gonadotropin-releasing hormone agonist-induced differences in granulosa cell cycle kinetics are associated with alterations in follicular fluid müllerian-inhibiting substance and androgen content. The Journal of Clinical Endocrinology & Metabolism, 76(3), 711-714. https://doi.org/10.1210/jcem.76.3.8445031
xxxiv Tal, R., & Seifer, D. B. (2017). Ovarian reserve testing: A user’s guide. American Journal of Obstetrics and Gynecology, 217(2), 129-140. https://doi.org/10.1016/j.ajog.2017.02.027
xxxv Overbeek, A., et al. (2012). Intra-cycle fluctuations of anti-müllerian hormone in normal women with a regular cycle: A re-analysis. Reproductive BioMedicine Online, 24(6), 664-669. https://doi.org/10.1016/j.rbmo.2012.02.023
xxxvi Sowers, M., et al. (2010). Anti-müllerian hormone and inhibin B variability during normal menstrual cycles. Fertility and Sterility, 94(4), 1482-1486. https://doi.org/10.1016/j.fertnstert.2009.07.1674
xxxvii Tal, R., & Seifer, D. B. (2017). Ovarian reserve testing: A user’s guide. American Journal of Obstetrics and Gynecology, 217(2), 129-140. https://doi.org/10.1016/j.ajog.2017.02.027
xxxviii Gordon, C. E., & Yanushpolsky, E. (2021). Anti-müllerian hormone: Current understanding and clinical use. Current Obstetrics and Gynecology Reports, 10(4), 61-70. https://doi.org/10.1007/s13669-021-00310-7
xxxix Majumder, K., et al. (2010). The use of anti-müllerian hormone and antral follicle count to predict the potential of oocytes and embryos. European Journal of Obstetrics & Gynecology and Reproductive Biology, 150(2), 166-170. https://doi.org/10.1016/j.ejogrb.2010.02.029
xl Broer, S. L., et al. (2013). Added value of ovarian reserve testing on patient characteristics in the prediction of ovarian response and ongoing pregnancy: An individual patient data approach. Human Reproduction Update, 19(1), 26-36. https://doi.org/10.1093/humupd/dms041
xli Tal, R., & Seifer, D. B. (2017). Ovarian reserve testing: A user’s guide. American Journal of Obstetrics and Gynecology, 217(2), 129-140. https://doi.org/10.1016/j.ajog.2017.02.027
xlii Majumder, K., et al. (2010). The use of anti-müllerian hormone and antral follicle count to predict the potential of oocytes and embryos. European Journal of Obstetrics & Gynecology and Reproductive Biology, 150(2), 166-170. https://doi.org/10.1016/j.ejogrb.2010.02.029
xliii Broer, S. L., et al. (2013). Added value of ovarian reserve testing on patient characteristics in the prediction of ovarian response and ongoing pregnancy: An individual patient data approach. Human Reproduction Update, 19(1), 26-36. https://doi.org/10.1093/humupd/dms041
xliv Tal, R., & Seifer, D. B. (2017). Ovarian reserve testing: A user’s guide. American Journal of Obstetrics and Gynecology, 217(2), 129-140. https://doi.org/10.1016/j.ajog.2017.02.027
xlv Majumder, K., et al. (2010). The use of anti-müllerian hormone and antral follicle count to predict the potential of oocytes and embryos. European Journal of Obstetrics & Gynecology and Reproductive Biology, 150(2), 166-170. https://doi.org/10.1016/j.ejogrb.2010.02.029
xlvi Tal, R., & Seifer, D. B. (2017). Ovarian reserve testing: A user’s guide. American Journal of Obstetrics and Gynecology, 217(2), 129-140. https://doi.org/10.1016/j.ajog.2017.02.027
xlvii Steiner, A. Z., et al. (2017). Association between biomarkers of ovarian reserve and infertility among older women of reproductive age. JAMA, 318(14), 1367. https://doi.org/10.1001/jama.2017.14588
xlviii Zarek, S. M., et al. (2015). Is anti-müllerian hormone associated with Fecundability? Findings from the eager trial. The Journal of Clinical Endocrinology & Metabolism, 100(11), 4215-4221. https://doi.org/10.1210/jc.2015-2474
xlix Tal, R., & Seifer, D. B. (2017). Ovarian reserve testing: A user’s guide. American Journal of Obstetrics and Gynecology, 217(2), 129-140. https://doi.org/10.1016/j.ajog.2017.02.027
l Tal, R., & Seifer, D. B. (2017). Ovarian reserve testing: A user’s guide. American Journal of Obstetrics and Gynecology, 217(2), 129-140. https://doi.org/10.1016/j.ajog.2017.02.027
li Penzias, A., et al. (2020). Testing and interpreting measures of ovarian reserve: A committee opinion. Fertility and Sterility, 114(6), 1151-1157. https://doi.org/10.1016/j.fertnstert.2020.09.134
lii Rasool, S., & Shah, D. (2017). Fertility with early reduction of ovarian reserve: The last straw that breaks the camel’s back. Fertility Research and Practice, 3(1). https://doi.org/10.1186/s40738-017-0041-1
liii Broekmans, F. J., et al. (2010). The antral follicle count: Practical recommendations for better standardization. Fertility and Sterility, 94(3), 1044-1051. https://doi.org/10.1016/j.fertnstert.2009.04.040
liv Broekmans, F., et al. (2006). A systematic review of tests predicting ovarian reserve and IVF outcome. Human Reproduction Update, 12(6), 685-718. https://doi.org/10.1093/humupd/dml034
lv Conti, M., & Chang, R. J. (2016). Folliculogenesis, ovulation, and Luteogenesis. Endocrinology: Adult and Pediatric, 2179-2191.e3. https://doi.org/10.1016/b978-0-323-18907-1.00125-6
lvi Scheffer, G., et al. (2003). The number of antral follicles in normal women with proven fertility is the best reflection of reproductive age. Human Reproduction, 18(4), 700-706. https://doi.org/10.1093/humrep/deg135
lvii Shahrokh Tehraninezhad, E., et al. (2016). Analysis of ovarian reserve markers (AMH, FSH, AFC) in different age strata in IVF/ICSI patients. International journal of reproductive biomedicine, 14(8), 501–506.
lviii Aiyappan, S. K, et al. (2016). Age-related Normogram for ovarian Antral follicle count in women with polycystic ovary syndrome and comparison with age matched controls using magnetic resonance imaging. Journal of Clinical and Diagnostic Research. https://doi.org/10.7860/jcdr/2016/17334.7136
lix Guo, Y., et al. (2021). Efficacy of three COS protocols and predictability of AMH and AFC in women with discordant ovarian reserve markers: A retrospective study on 19,239 patients. Journal of Ovarian Research, 14(1). https://doi.org/10.1186/s13048-021-00863-4
lx Guo, Y., et al. (2021). Efficacy of three COS protocols and predictability of AMH and AFC in women with discordant ovarian reserve markers: A retrospective study on 19,239 patients. Journal of Ovarian Research, 14(1). https://doi.org/10.1186/s13048-021-00863-4
lxi Sunkara, S. K., et al. (2011). Association between the number of eggs and live birth in IVF treatment: An analysis of 400 135 treatment cycles. Human Reproduction, 26(7), 1768-1774. https://doi.org/10.1093/humrep/der106
lxii Gunnala, V., et al. (2017). One thousand seventy-eight autologous IVF cycles in women 45 years and older: The largest single-center cohort to date. Journal of Assisted Reproduction and Genetics, 35(3), 435-440. https://doi.org/10.1007/s10815-017-1088-y
lxiii Sunkara, S. K., et al. (2011). Association between the number of eggs and live birth in IVF treatment: An analysis of 400 135 treatment cycles. Human Reproduction, 26(7), 1768-1774. https://doi.org/10.1093/humrep/der106
lxiv Gunnala, V., et al. (2017). One thousand seventy-eight autologous IVF cycles in women 45 years and older: The largest single-center cohort to date. Journal of Assisted Reproduction and Genetics, 35(3), 435-440. https://doi.org/10.1007/s10815-017-1088-y
lxv Van der Gaast, M., et al. (2006). Optimum number of oocytes for a successful first IVF treatment cycle. Reproductive BioMedicine Online, 13(4), 476-480. https://doi.org/10.1016/s1472-6483(10)60633-5
lxvi Session 32: Efficacy in ART, Human Reproduction, Volume 25, Issue suppl_1, June 2010, Pages i47–i49, https://doi.org/10.1093/humrep/de.25.s1.32
lxvii Sunkara, S. K., et al. (2011). Association between the number of eggs and live birth in IVF treatment: An analysis of 400 135 treatment cycles. Human Reproduction, 26(7), 1768-1774. https://doi.org/10.1093/humrep/der106
lxviii Fatemi, H. M., et al. (2012). High ovarian response does not jeopardize ongoing pregnancy rates and increases cumulative pregnancy rates in a gnrh-antagonist protocol. Human Reproduction, 28(2), 442-452. https://doi.org/10.1093/humrep/des389
lxix Steward, R. G., et al. (2014). Oocyte number as a predictor for ovarian hyperstimulation syndrome and live birth: An analysis of 256,381 in vitro fertilization cycles. Fertility and Sterility, 101(4), 967-973. https://doi.org/10.1016/j.fertnstert.2013.12.026
lxx Sunkara, S. K., et al. (2011). Association between the number of eggs and live birth in IVF treatment: An analysis of 400 135 treatment cycles. Human Reproduction, 26(7), 1768-1774. https://doi.org/10.1093/humrep/der106
lxxi Magnusson, Å., et al. (2017). The number of oocytes retrieved during IVF: A balance between efficacy and safety. Human Reproduction, 33(1), 58-64. https://doi.org/10.1093/humrep/dex334
lxxii Siddhartha, N., et al. (2016). Correlation of serum estradiol level on the day of ovulation trigger with the reproductive outcome of intracytoplasmic sperm injection. Journal of Human Reproductive Sciences, 9(1), 23. https://doi.org/10.4103/0974-1208.178631
lxxiii Revelli, A., et al. (2014). A critical review of Bi-dimensional and three-dimensional ultrasound techniques to monitor follicle growth: Do they help improving IVF outcome? Reproductive Biology and Endocrinology, 12(1), 107. https://doi.org/10.1186/1477-7827-12-107
lxxiv Abbara, A., et al. (2018). Follicle size on day of trigger most likely to yield a mature oocyte. Frontiers in Endocrinology, 9. https://doi.org/10.3389/fendo.2018.00193
lxxv Rosen, M., et al. (2008). A quantitative assessment of follicle size on oocyte developmental competence. Fertility and Sterility, 90(3), 684-690. https://doi.org/10.1016/j.fertnstert.2007.02.011
lxxvi Abbara, A., et al. (2018). Follicle size on day of trigger most likely to yield a mature oocyte. Frontiers in Endocrinology, 9. https://doi.org/10.3389/fendo.2018.00193
lxxvii Mohr-Sasson, A., et al. (2020). The association between follicle size and oocyte development as a function of final follicular maturation triggering. Reproductive BioMedicine Online, 40(6), 887-893. https://doi.org/10.1016/j.rbmo.2020.02.005
lxxviii Wirleitner, B., et al. (2018). Relationship between follicular volume and oocyte competence, blastocyst development and live-birth rate: Optimal follicle size for oocyte retrieval. Ultrasound in Obstetrics & Gynecology, 51(1), 118-125. https://doi.org/10.1002/uog.18955
lxxix Wirleitner, B., et al. (2018). Relationship between follicular volume and oocyte competence, blastocyst development and live-birth rate: Optimal follicle size for oocyte retrieval. Ultrasound in Obstetrics & Gynecology, 51(1), 118-125. https://doi.org/10.100 2/uog.18955
lxxx Shapiro, B. S., et al. (2022). The effect of ovarian follicle size on oocyte and embryology outcomes. Fertility and sterility, 117(6), 1170–1176. https://doi.org/10.1016/j.fertnstert.2022.02.017
lxxxi Wirleitner, B., et al. (2018). Relationship between follicular volume and oocyte competence, blastocyst development and live-birth rate: Optimal follicle size for oocyte retrieval. Ultrasound in Obstetrics & Gynecology, 51(1), 118-125. https://doi.org/10.1002/uog.18955
lxxxii Wirleitner, B., et al. (2018). Relationship between follicular volume and oocyte competence, blastocyst development and live-birth rate: Optimal follicle size for oocyte retrieval. Ultrasound in Obstetrics & Gynecology, 51(1), 118-125. https://doi.org/10.1002/uog.18955
lxxxiii Wirleitner, B., et al. (2018). Relationship between follicular volume and oocyte competence, blastocyst development and live-birth rate: Optimal follicle size for oocyte retrieval. Ultrasound in Obstetrics & Gynecology, 51(1), 118-125. https://doi.org/10.1002/uog.18955
lxxxiv Triwitayakorn, A., et al. (2003). Correlation between human follicular diameter and oocyte outcomes in an ICSI program. Journal of assisted reproduction and genetics, 20(4), 143–147. https://doi.org/10.1023/a:1022977002954
lxxxv Xiao, Y., et al. (2018). Follicular Flushing increases the number of oocytes retrieved in poor ovarian responders undergoing in vitro fertilization: A retrospective cohort study. BMC Women's Health, 18(1). https://doi.org/10.1186/s12905-018-0681-2
lxxxvi Aktas, M., et al. (2005). Oocytes in the empty follicle: A controversial syndrome. Fertility and Sterility, 84(6), 1643-1648. https://doi.org/10.1016/j.fertnstert.2005.05.060
lxxxvii Coulam, C. B., et al. (1986). Empty follicle syndrome. Fertility and Sterility, 46(6), 1153-1155. https://doi.org/10.1016/s0015-0282(16)49898-5
lxxxviii Deepika, K., et al. (2015). Empty follicle syndrome: Successful pregnancy following dual trigger. Journal of Human Reproductive Sciences, 8(3), 170. https://doi.org/10.4103/0974-1208.165152
lxxxix Revelli, A., et al. (2014). A critical review of Bi-dimensional and three-dimensional ultrasound techniques to monitor follicle growth: Do they help improving IVF outcome? Reproductive Biology and Endocrinology, 12(1), 107. https://doi.org/10.1186/1477-7827-12-107
xc Revelli, A., et al. (2017). Empty follicle syndrome revisited: definition, incidence, aetiology, early diagnosis and treatment. Reproductive Biomedicine Online 35(2), 132-138. https://doi.org/10.1016/j.rbmo.2017.04.012